Neuroscience
Rhawn Gabriel Joseph, Ph.D.
Brain Research Laboratory
ABSTRACT
The human brainstem is fashioned around the 7th week of gestation and matures in a caudal to rostral arc thereby forming the medulla, pons, and midbrain. The medulla mediates arousal, breathing, heart rate, and gross movement of the body and head, and medullary functions appear prior to those of the pons which precede those of the midbrain. Hence, by the 9th gestational week the fetus will display spontaneous movements, one week later takes its first breath, and by the 25th week demonstrates stimulus-induced heart rate accelerations. As the pons, which is later to mature, mediates arousal, body movements, and vestibular and vibroacoustic perception, from around the 20th to 27th weeks the fetus responds with arousal and body movements to vibroacoustic and loud sounds delivered to the maternal abdomen. The midbrain inferior-auditory followed by the superior-visual colliculi is the last to mature, and in conjunction with the lower brainstem makes fine auditory discriminations, and reacts to sound with fetal heart rate (FHR) accelerations, head turning, and eye movements--around the 36th week. When aroused the fetus also reacts with reflexive movements, head turning, FHR accelerations, and may fall asleep and display rapid eye movements. Thus fetal-cognitive motor activity, including auditory discrimination, orienting, the wake-sleep cycle, FHRs, and defensive reactions, appear to be under the reflexive control of the brainstem which also appears capable of learning-related activity.
It is now well established that the human fetus is capable of some degree of behavioral complexity. In fact, as early as the 9th week of gestation the fetus is able to spontaneously move the extremities, head, and trunk (de Vries, Visser, & Prechtl, 1985). It has also been suggested that the near term fetus may be endowed with some degree of cognitive capability (e.g., Hepper & Shahidullah, 1994; Kisilevsky, Fearson & Muir, 1998). Cognition has been inferred based on alterations in fetal heart rate (FHR) and habituation to airborne sound (Kisilevsky & Muir, 1991), response-declines to vibroacoustic stimuli (Kisilevsky et al., 1998; Kuhlman, Burns, Depp, & Sabagha, 1988), and what appears to be neonatal preferences for the maternal voice as well as melodies and stories presented up to six weeks prior to birth (DeCasper & Fifer, 1980; DeCasper & Spence, 1986; DeCasper, et al. 1994; Lecanuet, et al. 1989).
As will be detailed below, the behavior of the fetus and newborn is likely a reflection of reflexive brainstem activities which are produced in the absence of forebrain-mediated affective or cognitive processing, i.e. thinking, reasoning, understanding, or true emotionality (Joseph, 1996a, 1999; Levene, 1993; Sroufe, 1996). It is the much slower to develop forebrain which generates higher order cognitive activity and purposeful behaviors, and which is responsible for the expression and experience of true emotions including pleasure, rage, fear and joy and the desire for social-emotional contact (Joseph, 1992, 1996ab, 1999; MacLean, 1990).
At birth and for the ensuing weeks, the forebrain is so immature that its influences are limited to signaling distress in reaction to hunger or thirst; a function of the immature hypothalamus (Joseph, 1982, 1992, 1999) in conjunction with the midbrain periaqueductal gray (e.g. Larson, Yajima, & Ko, 1994; Zhang, Davis, Bandler, & Carrive, 1994). Although various limbic nuclei become functionally mature over the course of the first several postnatal months and years (Benes, 1994; Joseph, 1992, 1999), the neocortex and lobes of the brain take well over seven, ten, and even thirty years to fully develop and myelinate (Blinkov & Glezer, 1968; Conel, 1939, 1941; Flechsig, 1901; Huttenlocher, 1990; Yakovlev & Lecours, 1967).
It is rather obvious that the neonate is able to scream and cry and can even slightly lift the corners of the mouth as if smiling. However, these do not appear to be true emotions (Sroufe, 1996; however, see Izard, 1991). In fact, smiling, as well as screaming and crying can be produced from brainstem stimulation even with complete forebrain transection or destruction (Larson et al., 1994; Zhang et al., 1994; reviewed in Joseph, 1996a). Hence, neonatal and premature infant "smiling" or distress reactions to noxious stimulation (e.g. heel lance) are also likely brainstem mediated, particularly in that they may be triggered in the absence of any obvious stimulus source and following forebrain destruction or lack of development (anencephaly). However, as brainstem maturation continues in a caudal-rostral arc (Debakan, 1970; Langworthy, 1937), at term and over the following weeks and months, the immature hypothalamus (which sits atop the midbrain), and thus the forebrain, increasingly contributes to and gains control over these behaviors (Joseph, 1992, 1999).
The progression in behavioral complexity that begins with spontaneous fetal movements and which culminates with presumed preferences for the sound of mother's voice, also appear to reflect maturational events taking place in the brainstem, followed by forebrain structures. Indeed, the brainstem is first fashioned around the 33rd day of gestation (Bayer, 1995; Marin-Padilla, 1988; Sidman & Rakic, 1982) and nearly completes its cycle of development and myelination around the 7th gestational month (Gilles, Leviton, & Dooling, 1983; Langworthy, 1937; Yakovlev & Lecours, 1967). However, in contrast to the forebrain, the brainstem is incapable of cognition such as reasoning, comprehension, or thought (Joseph, 1996c), but instead reflexively reacts to a variety of stimuli in an exceedingly complex, albeit stereotyped fashion (Blessing, 1997; Cohen, Rossignol & Gillner, 1988; Cowie, Smith, & Robinson,1994; Steriade & McCarley, 1990).
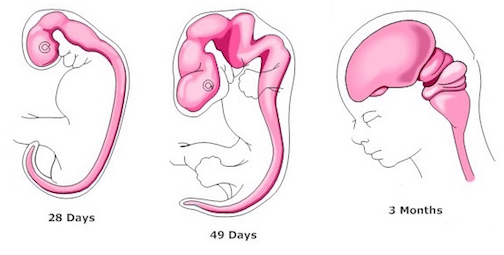
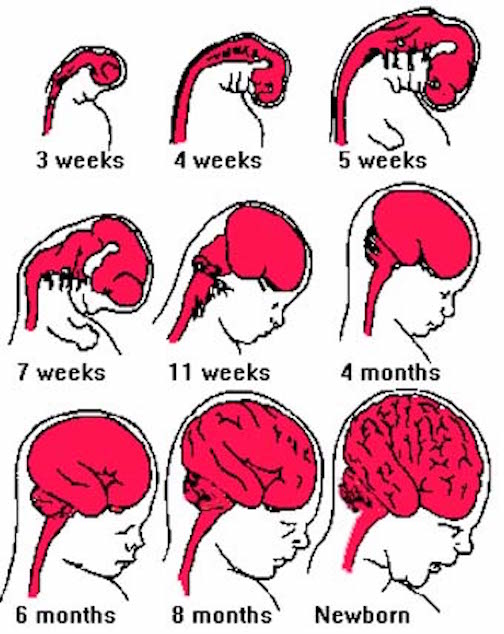
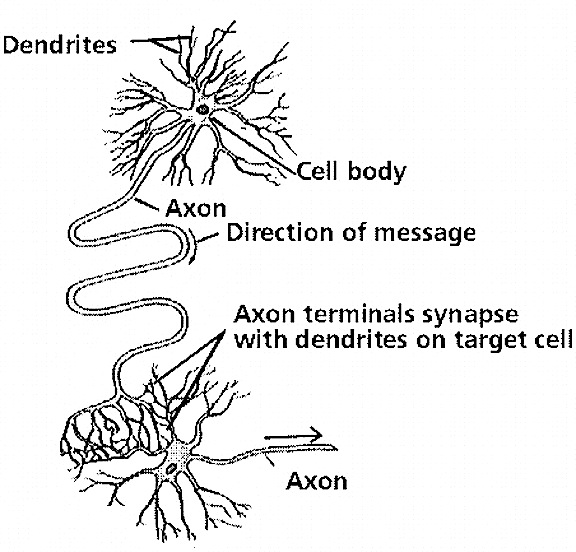
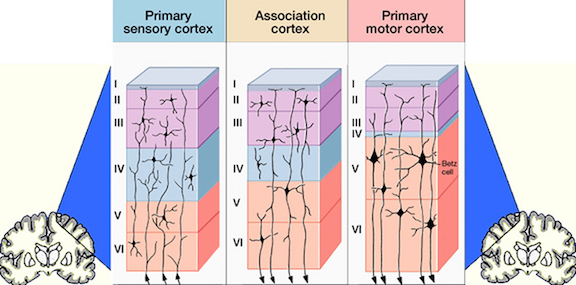
Fetal brain development can be divided into ten stages (Joseph, 1996a)
1. The generation of the neuroectoderm from ectoderm, and the formation of the neural preplate.
2. The splitting of the preplate thereby forming the neural plate.
3. The rising up and the inward folding of the sides of the neural plate which arch together and create a neural tube.
4. The generation of neuroepithelium which gives birth to immature nerve cells, the neuroblasts which migrate and grow leading axonal appendages, and then aggregate in specific, genetically determined locations beginning with what will become the brainstem and spinal cord.
5. The division of the neuroepithelium into ventricular and subventricular zones which produce separate waves of migrating neuroblasts.
6. Flexure of the neural tube which ascends, twists, and bends due to continued neuroblast generation and neuron division, thereby forming three (medulla, pons, forebrain), then later two additional cerebral vesicles (midbrain, telencephalon).
7. The aggregation, differentiation, and division of neurons which form the nuclei of the medulla, then the pons, midbrain, hypothalamus, thalamus, and later the limbic system, striatum, and later, layers I and VII (VIa,b) of the neocortex.
8. The establishment of neocortical layers II through VI as successive waves of neuroblasts are generated and form columns and layers as they sandwich themselves between neocortical layers I and VII (VIa,b).
9. Neuronal differentiation, dendritic growth, the establishment of synapses, and the myelination of activated brainstem axons which have established dendritic synaptic connections.
10. Functional development of the medulla, followed by the pons, then the midbrain, the lastly, the forebrain which does not begin to functionally mature until near term.
THE BRAINSTEM: A FUNCTIONAL OVERVIEW
The brainstem consists of phylogenetically older neurons and nuclei and is organized in a somewhat similar manner across a host of species ranging from fish to woman and man (Aitkin, 1986; Joseph, 1996c; Vertes, 1990) The brainstem is an exceedingly complex structure, consisting of a variety of nuclei and subdivisions which perform an array of divergent as well as interrelated sensory and reflexive motor functions (Blessing, 1997; Klemm, 1990; Skinner & Garcia-Rill, 1990; Vertes, 1990). These include the mediation and control of arousal, orienting, the sleep cycle, heart rate, breathing, gross axial, limb, head and eye movement (Blessing, 1997; Cowie et al., 1994; Masino, 1992; Steriade & McCarley, 1990), as well as visual, somesthetic, gustatory, and acoustic perception and sound production such as screaming and crying (Aitkin, 1986; Davidson & Bender,1991; Larson & Yajima, 1994; Zhang et al., 1994).
Given its exceedingly long and ancient evolutionary history, not surprisingly, many brainstem functions are present before birth and occur without the aid of thinking, reasoning, or even forebrain/neocortical participation (Blessing, 1997; Joseph, 1996cd; Steriade & McCarley, 1990). That is, the motor programs which subserve many basic and vital functions, such as the regulation of heart rate, the sleep cycle, and respiration, are essentially genetically hardwired, reflexively initiated, and produced in accordance with the brainstem's synaptic organization and internally generated rhythms which have been acquired and molded over the course of evolution. Because so many brainstem functions occur in a rhythmic, diurnal, and/or reflexive fashion, they do not require the assistance of the forebrain (Blessing, 1997; Cohen et al., 1988; Joseph, 1996c; Klemm, 1990; Steriade & McCarley, 1990).
Indeed, these same reflexive and rhythmic activities are demonstrated by anencephalic infants who may possess only a brainstem, i.e. respiration, sleeping, waking, crying, leg kicking, rudimentary smiling, and even rapid eye movements while sleeping. Rather, it is only later life that the maturing forebrain begins to exert significant influence on brainstem functioning.
By the 7th gestational month the medulla and pons have nearly completed their cycle of myelination and most of the various descending spinal-motor fiber tracts have reached target tissues and established their synaptic interconnections (Gilles et al., 1983; Langworthy, 1937; Yakovlev & Lecours, 1967). However, because the fetal brainstem matures in a caudal to rostral arc, and as different nuclei mature and myelinate at different rates, fetal-brainstem reflexes are initially triggered infrequently or in isolation, and thus emerge gradually and in an irregular fashion (Debakan, 1970).
For example, around the 10th week of gestation the fetus may take a single "breath" over a 24 hour time period, whereas by the 40th week "breathing" occurs much more frequently with some degree of regularity in regard to chest and abdominal movement (de Vries et al., 1985; Natale, Nasello-Paterson & Connors, 1988). However, it's not until birth that the breathing (inhalation - exhalation) response occurs in a continual fashion; a function of increasingly mature brainstem development.
Nevertheless, the brainstem continues to mature well after birth, and correspondingly, brainstem reflexes emerge and disappear at different time periods over the course of the first three to six months of postnatal life (Debakan, 1970; see also Capute, Palmer, Accardo, Wachtel, Ross & Palmer, 1984; Piper & Darrah, 1994). For example, initially, vital functions such as heart rate and respiration are irregular, body temperatures fluctuate, and swallowing is precarious. In addition, reflexes such as sneezing, yawning, sweating, salivation, and urination are almost hypersensitive and easily triggered. Moveover, similar to those with forebrain destruction or transection, newborn infants can make stepping, walking, running, crawling and even swimming movements. Neonates can also involuntary grope and reflexively grasp with their hands. These same behaviors are also demonstrated by anencephalics.
Over the ensuing months these reflexes become subject to increasing neurological maturational control and either become stable or incorporated and subsumed by the forebrain, or they disappear and are suppressed; albeit at different time periods in accordance with the maturational events still taking place throughout the brainstem as well as the forebrain. However, if the forebrain were destroyed many of these same primitive reflexes will reappear, including the sucking, head turning, groping, grasping, tonic neck, labyrinthine, supporting, placing, and stepping reflexes.
THE DEVELOPMENT OF THE MEDULLA: A FUNCTIONAL OVERVIEW
Broadly considered, the brainstem consists of the medulla, pons, and midbrain, and matures in a caudal to rostral arc--a process that begins around the 6th to 7th week of gestation and continues well into the first year of postnatal life (Debakan, 1970; Gilles et al., 1983; Sidman & Rakic, 1982; Yakovlev & Lecours, 1967). Hence, the nuclei of the medulla generally emerge and myelinate prior to those of the pons which precede those of the midbrain. As noted above, this pattern of functional development is also demonstrated behaviorally in the fetus and neonate.
For example, in addition to its many subnuclei, the medulla gives rise to a variety of descending spinal-motor tracts which reflexively trigger limb and body movement. It also sprouts five cranial nerves, the acoustic (VIII), glossopharyngeal (IX), vagus (X), accessory (XI) and hypoglossal (XII), which exert tremendous influences on gross body movement, heart rate, respiration, and head turning. Specifically, the hypoglossal nerve and nucleus, controls the tongue and influences body movement. The spinal accessory nerve and nucleus controls shoulder elevation and head turning. The vagus, glosopharyngeal nerve, and nucleus solitarious control respiration and heart rate.
In regard to heart rate, the nucleus of the solitary tract interacts with and is also coextensive with the medullary respiratory and vasomotor centers. Together with the vagus and glosopharyngeal nerve and in conjunction with the "autonomic nervous system" these nuclei act to excite and depress cardiovascular functioning (Aminoff, 1996; Blessing, 1997; Joseph, 1996c). As per the medulla, control over the cardiovascular system is accomplished via pre- and post-ganglionic vagal fibers which project to the atrial muscle and the sinoatrial and atrioventicular nodes of the heart. However, in regard to fetal development and heart rate, the differential developmental rates of the parasympathetic system vs the sympathetic system, also appear to exert significant influences on the different maturational patterns of FHR deceleration and acceleration (see Kisilevsky & Low, 1998; Sorokin, et al. 1982; Wheeler & Murrills, 1978).
As the medullary reticular formation constitutes the core of the medulla this portion of the brainstem is also able to stimulate and thus activate the spinal cord, as well as the more rostral portions of the brain including the forebrain and neocortex (Blessing, 1997; Steriade & McCarley, 1990). However, as the medulla matures in advance of more rostral structures, reflexive movements of the head, body, extremities, as well as "breathing" movements and alterations in heart rate, appear in advance of other functions.
SPONTANEOUS HEART RATE CHANGES, BODY AND BREATHING MOVEMENTS
The neural tube is first fashioned three weeks after conception and by the 7-8th week of gestation the major structures of the medulla have been established (Gilles et al., 1983; Sidman & Rakic, 1982). These include the hypoglossal, spinal accessory, vagus, and glosopharyngeal cranial nuclei, and the neurons of the reticular activating system. As noted, these nuclei and activating pathways subserve arousal, generalized body movements, head turning, shoulder elevation, heart rate, and breathing.
Likewise, beginning as early as the 7th week of gestation, the infant will display spontaneous movements, which by the 9th week come to include the extremities, the trunk, and the head (de Vries et al., 1985). Soon thereafter the fetus will take its first "breath" and by the 10th week of gestation spontaneous "breathing" (chest and abdominal) movements are observed (de Vries et al.,1985).
Initially these "breathing" movements remain rather irregular, variable, and isolated events, such that perhaps one breath might be observed over the course of an hour. Over the ensuing weeks and months these breathing movements occur more frequently and by 24 weeks they may be observed about 14% of the time. By 40 weeks the fetus will breath about 30% of the time over a 24 hour period (de Vries et al. 1985; Natale et al., 1988; Patrick, Campbell, Carmichael, Natale, & Richardson,1980).
As there is no free oxygen which may be inhaled, these "breathing" movements are brainstem reflexes which tend to be produced during periods of brainstem activity and heightened arousal (e.g., Pillai & James, 1990). Thus the aroused fetus (Pillai & James, 1990; Sorokin et al., 1982) not only will take a "breath" but will demonstrate alterations in heart rate and spontaneous movements which are also produced secondary to high levels of brainstem activity --as it is the brainstem which mediates arousal. Thus, during high levels of brainstem arousal, the fetus will spontaneously move its head, trunk, and extremities (de Vries et al., 1985), whereas heart rate may dramatically accelerate (Sorokin et al., 1982).
Moreover, just as breathing occurs more frequently as the medulla matures, spontaneous movements are observed more frequently as well. By 10 weeks spontaneous body movements occur about 14% of the time, whereas by 19 weeks the fetus is active more than 50% of the time (de Vries et al., 1985). That does not mean that FHR acceleration, breathing, and body movements are strictly coupled. For example, after 20 weeks there is an increase in the percentage of FHR acceleration and movement (DiPietro, Hodgson, Costigan, Hilton, & Johnson, 1996), until around 30 weeks at which point body movements decline (Natale, Nasello-Paterson, & Turlink, 1985). By contrast, a decrease in fetal "breathing" is not observed until two days before labor onset (Patrick et al., 1980), which, however, may be due to mechanisms initiating labor rather than maturation per se.
By term, heart rate fluctuations and accelerations again occur in tandem with spontaneous body movements and heightened arousal (Timor-Tritch, Dierker, Hertez, Deagan, & Rosen, 1978); a likely function of maturational changes occuring within the medulla and throughout the brainstem.
However, just as heightened brainstem activity is associated with labored breathing, but a general lack of movement during "paradoxical" sleep (Steriade & McClure, 1990), alterations in brainstem arousal may produce fetal "breathing" but a lack of movement during "quiet" states. As in "deep sleep," "quiet" periods may be due to heightened brainstem inhibitory influences over the spinal cord and cranial nerves; for electrophysiologically, during "deep sleep," the brain is in fact highly active (Steriade & McClure, 1990). Hence the term "paradoxical" sleep; a seemingly "quiet" period associated with a lack of limb, head, or whole body movement other than jerks and twitches, lateral eye movements, and labored breathing.
That these fetal reflexes are produced by an immature and still maturing brainstem and do not reflect cognitive processing or purposeful movement activity is suggested by the fact that breathing and spontaneous movements actually decrease as the fetus nears term (Kozuma, Nemota, Okal, & Mizuno, 1991; Patrick et al., 1980; Patrick, Campbell, Carmichael, Natale, & Richardson, 1982; Trudinger, Aust, & Knight, 1985), though again the contributions of mechanisms related to labor initiation cannot be ruled out. Thus, as the medulla myelinates, stabilizes, and matures, these spontaneous reflexes are not as easily triggered, but begin to be governed by the more stable intrinsic activities generated within the brainstem. Hence, at birth the infant takes its first real breath which is then sequentially repeated without interruption. Likewise, as the brainstem continues to mature after birth, brainstem regulated functions, such as heart rate and breathing, become increasingly stabilized over the ensuing weeks and months.
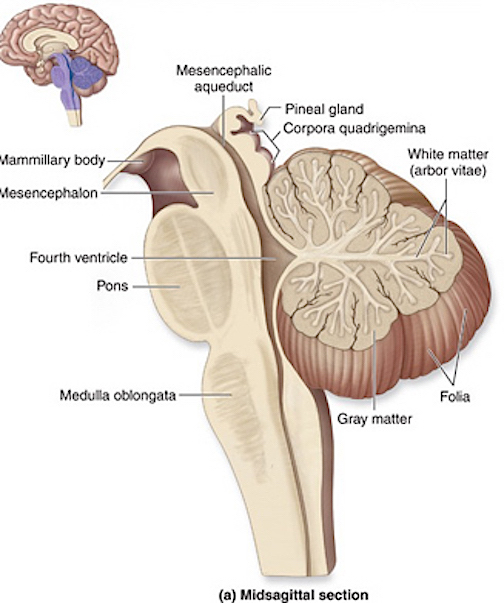
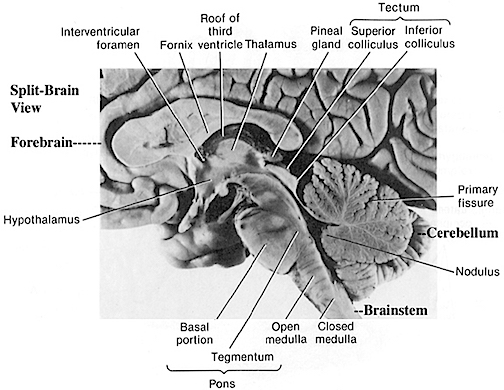
THE DEVELOPMENT OF THE PONS: A FUNCTIONAL OVERVIEW
Like the medulla, the organization of the pons is neuroanatomically and physiologically complex and consists of a number of specialized as well as diffusely acting nuclei. As the pons is coextensive with the medulla, medullary fiber pathways, including the rostral continuation of the medullary reticular formation, course through its core (Blessing, 1997; Steriade & McCarley, 1990; Vertes, 1990).
The structures of the pons include the trigeminal (V), abducens (VI), facial (VII), and vestibulocochlear cranial nerves (VIII), the medial longitudinal fasciculus (MLF), and the pontine tegmentum, raphe nucleus, and locus coeruleus which exert widespread influences on arousal including the sleep-wake cycle (Klemm, 1990; Steriade & McCarley, 1990; Vertes, 1990). The fifth and seventh nerves, of course, are concerned with facial sensation and movement, whereas the vestibulocochlear nerve and the auditory pathways respond selectively to vibration, loud sounds and specific frequencies within the auditory spectrum. Because the MLF is interconnected with these nuclei and also descends through the medulla and into the spinal cord where it establishes synaptic contact with medullary and spinal motor neurons, high levels of pontine arousal including the perception of loud sounds can trigger head turning, whole body movement and the startle reaction in the near term fetus, and the Moro reflex in the neonate.
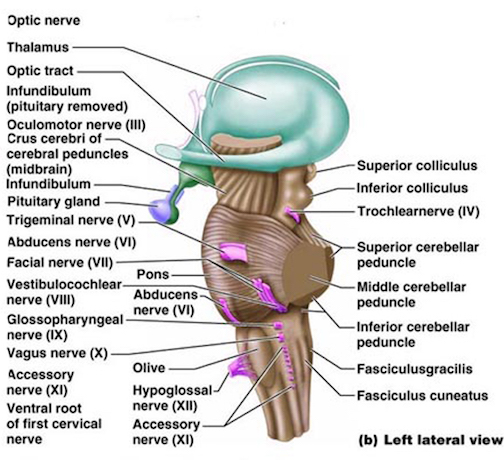
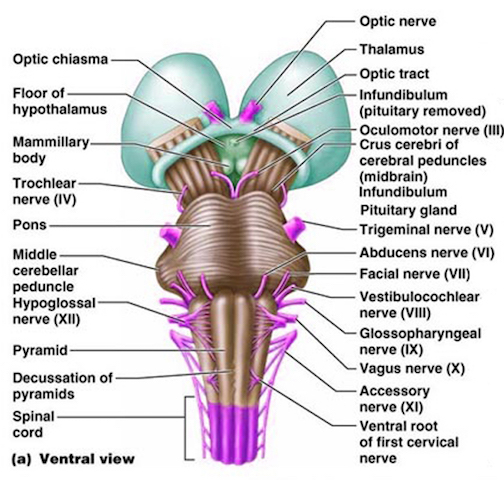
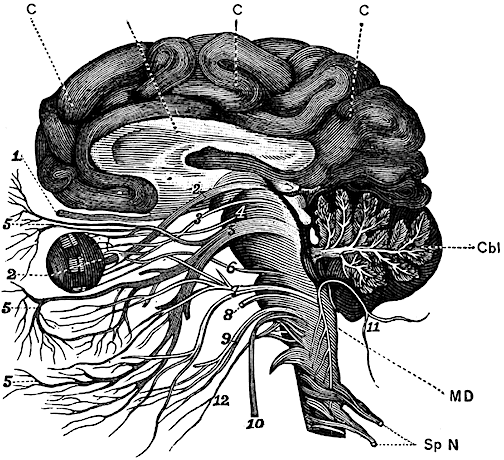
VIBRATORY-ACOUSTIC PERCEPTION
As noted, the brainstem matures in a caudal to rostral direction, and the pons begins to emerge after the medulla, around the 8th week of gestation. The maturation and myelination of the pons is more prolonged than the medulla and does not reach advanced levels of development until around the 7th month of gestation (Langworthy, 1937; Yakovlev & Lecours, 1967). Hence, many of the functions associated with the pons appear later in fetal development; i.e. around the 7th month of gestation. As with the medulla, some functions appear in advance of others and in an incomplete or irregular fashion.
For example, it has been reported that by the 26th week of gestation the body of the fetus will reflexively react to vibration and exceedingly loud auditory (110 db) stimulation 60% of the time, whereas by 32 weeks the rate is over 80% (Kisilevsky, Muir & Low, 1992; Shahidullah & Hepper, 1993). At 29 weeks not only will the body jerk, but the FHR will accelerate.
As the brainstem continues to mature it becomes increasingly responsive to external sounds. By the 36th to 38th week of gestation, the fetal brainstem will respond to external noises and even the sound of mother's voice with reflexive body movements, head turning, and FHR acceleration, albeit, about 30% to 60% of the time (Lecanuet et al. 1995; Schmidt, et al. 1985). Again, these sounds must be high frequency and quite loud as uterine attenuation may be as high as 70 db (Querleu et al 1986), though more recent work suggests that maximum attenuation is about 40 db (Peters & Abrams, 1993; Richards, et al.1992).
Moreover, these sounds tend to trigger body movements or heart rate acceleration when the fetus is already highly aroused (Schmidt et al., 1985). Once aroused, early maturing brainstem reflexes are easily triggered. Again, however, it must be emphasized that just as lateral and conjugate eye movements are initiated and breathing and heart rate may accelerate during deep "paradoxical" sleep, and just as an adult in "quiet" (slow-wave) sleep may be easily aroused, the fetal brainstem may also react to stimulation with FHR accelerations and movement during quiet periods.
Via the 8th nerve and cochlear nucleus, the pons (in conjunction with the medulla and midbrain) is capable of responding to and differentiating between specific auditory frequencies (Aitkin, 1986; Klemm, 1990; Vertes, 1990). Indeed, the cochlear nucleus is tonotopically organized such that specific neurons react to specific sounds. Hence, as the pons continues to mature, and by the 36th week of gestation, the fetus appears able to attend to and may be able to discriminate between different sounds as measured by heart rate decelerations (Lecanuet, Granier-Deferre, Jacquet & Busnel, 1992). The implications of these latter results, however, are open to question as the reported decelerations were rather small (0.4 to 0.8 BPM).
ENVIRONMENTAL FINE TUNING OF THE BRAINSTEM
As the brain is formed, billions of excess neurons and dendrites are produced which in turn form innumerable random interconnections (Rakic, Bourgeois, Echenhoff, Zaecevic & Goldman-Rakic, 1986). Over the course of late fetal development and over the first several years of life, billions of these excess dendrites, synapses, and neurons are absorbed, discarded and die (Joseph, 1982, 1998). This process of elimination and programmed cell death is also under environmental control (e.g., Casagrande & Joseph, 1978. 1980; Joseph, 1999). That is, the maturing, developing brain sheds innumerable excess neurons, dendrites, and random synaptic interconnections, as a function of experience, or lack thereof.
Although neurons continue to be generated and to divide during adulthood, it is through environmentally sculpted attrition and dendritic pruning that specific neural networks are established and formed. The sculpting of specific neural pathways fine tunes perception and selective attention, and promotes learning, memory, and language, cognitive and personality development (Joseph, 1982, 1996b, 1999).
Brainstem nuclei and pathways also display synaptic plasticity, and in this regard can be influenced by auditory and visual experience and are capable of synaptic "learning" as represented by collateral axonal and dendritic sprouting and alterations in neural response properties (Isukahara, 1985; Moore & Aitkin, 1975; Moore & Irvine, 1981). For example, through repetitive auditory stimulation, or lack thereof, specific midbrain neural networks and pathways are molded, and these, in turn, selectively respond to the stimuli which shaped them (Moore & Aitkin, 1975; Moore & Irvine, 1981).
Moreover, as has been demonstrated in various avian species, during early development selective exposure to certain sounds and songs, such as those of other birds, can induce a selective preference for attending to and producing them (Konishi, 1974; Stevenson, 1974; Thorpe, 1974). Hence, since the fetal and neonatal brainstem is capable of perceiving sound it increasingly becomes responsive to those sounds which it is repeatedly exposed to. Conversely, those neural pathways which fail to be stimulated drop out and die.
Likewise, DeCasper, Lecanuet, and colleagues have provided some intriguing evidence which suggests that the brain of the human fetus can become organized so as to "recognize" or selectively respond, at term, to its mother's voice and particular melodies, or other familiar or repetitive sounds presented up to six weeks before birth. This has been demonstrated through preference tests using a nonnutritive sucking paradigm and heart rate deceleration mesures (DeCasper & Fifer, 1980; DeCasper & Spence, 1986; DeCasper et al., 1994; Lecanuet et al., 1989). In this regard, the fetal brain appears capable of generating and supporting what might best be described as rudimentary learning-related activity.
Nevertheless, although the brainstem can be trained to react to specific sounds does this imply cognition, as in thinking or reasoning? Is there any evidence that the fetus (or neonate) recognizes and thus "knows" what he or she may be hearing, or is this a form of neural conditioning? Although the fetal brain appears to be capable of "classical" habituation (Kisilevsky & Muir, 1991) and presumably dishabituation, does this imply that the fetus is reacting to the stimulus as meaningful, or in a controlled purposeful manner? Even an isolated neuron placed in a saline solution can display habituation and neural plasticity, and in this regard habituation/dishabituation may represent little more than neural fatigue followed by recovery (Schmidt, 1978). These are physiological, reflexive responses which do not necessarily imply cognition.
On the other hand, it could also be argued that since the fetal brainstem appears capable of learning, that this, in-itself is evidence for rudimentary cognitive-like (i.e. learning-related) activity.
THE DEVELOPMENT OF THE MIDBRAIN: A FUNCTIONAL OVERVIEW
The midbrain is the least differentiated segment of the brainstem, and consists of the dopamine producing substantia nigra, the inferior-auditory and superior-visual colliculus, and the trochlear (IV) and oculomotor (III) cranial nerves which, in conjunction with the MLF and abducens (VI) control eye movements (Aitkin, 1986; Vertes, 1990). The midbrain is actually coextensive with the pons and these structures interact and share overlapping nuclei and coextensive pathways, such as the MLF and reticular core, which, in the midbrain becomes the tegmentum--the rostral extension of the pontine-medullary reticular formation.
The caudal to rostral pattern of brainstem maturation is also characteristic of the midbrain with the inferior-auditory colliculus beginning its cycle of maturation around the 6th week of gestation vs the 8th week for the superior-visual colliculus (Bayer et al., 1995; Sidman & Rakic, 1982). However, the cycle of development is rather prolonged as the inferior colliculus does not completely take form until around the 17th week, with the superior colliculus becoming established several weeks later. Moreover, it is not until well after birth that midbrain development and myelination is nearly complete (Debakan, 1970; Langworthy 1937; Yakovlev & Lecours, 1967).
Whereas the superior colliculus responds and orients to visual stimuli, the inferior colliculi is organized to react to sound. These midbrain structures generate high levels of arousal when stimulated, and trigger orienting responses by turning the head and initiating eye movements. Hence, as the fetal midbrain matures the fetus not only becomes increasingly responsive to sound but will reflexively orient, turn the head, and react with lateral eye movements if sufficiently stimulated and when highly aroused. However, in the neonate (Emde & Koenig, 1969; Emde & Metcalf, 1970), when highly aroused the stereotypical reaction of the brainstem as a whole is to fall asleep and produce rapid (lateral) eye movements (REM). Similar arousal-induced sleep reactions coupled with REM are likely characteristic of the late term fetus.
Lateral and horizontal eye movements are controlled by the oculomotor, trochlear, and abducens cranial nerves and the MLF, with the oculomotor nerve also mediating the pupillary reflex. As the brainstem develops and matures in a caudal to anterior direction, the abducens, which controls lateral eye movements, matures before the oculomotor and trochlear nerves, that is, around the 7th to 8th week of gestation (Langworthy 1937). By the 9th week, the oculomotor and trochlear cranial nerves have emerged and neurons which will form the inferior and superior colliculus have been generated.
As noted, midbrain development is rather prolonged and its cycle of myelination is not complete until well after birth (Debakan, 1970; Langworthy 1937; Yakovlev & Lecours, 1967). Likewise, the fetal capacity to produce eye movement does not appear until late in gestation, just prior to term, around the 38th week (Nijhuis, Prechtl, Martin, & Bots, 1982). Presumably, the late development of the visual pathways is a function of the experience-expectant visual needs of its developing neurons, and the fact that prior to birth there is little to see, other than perhaps exceedingly filtered light passing through the abdominal wall. Although the fetus may in fact be exposed to varying degrees of light and shadow, this form of stimulation does not promote visual perceptual or neurological development (Joseph & Casagrande, 1980; Casagrande & Joseph, 1978, 1980).
In fact, as demonstrated in young primates, when visual perception is limited to filtered light and shadows, for example, following monocular lid-suture, once the sutures are removed and the eye is opened, these animals not only react as if blind, but those neurons which normally receive this input, shrink and become non-functional (Joseph & Casagrande, 1980; Casagrande & Joseph, 1978, 1980). Thus, although the fetal eyes may open and the fetus may display a startle-blink as early as 24 weeks (Birnholz & Benacerraf, 1983), filtered light stimulation does not promote visual development. Fetal eye movements and blinking are brainstem reflexes.
In fact, due in part to midbrain and visual pathway immaturity (as well as numerous other factors), even if the fetus, that is, a premature infant, is provided "normal" visual stimulation, this input does not promote visual development. Rather, the development of and capacity to perceive, visually inspect, or discriminate visual patterned stimuli is disrupted and slowed as compared to full-term infants (Rose, Feldman, McCarton, & Wolfson, 1988).
In conclusion, it appears that these initial lateral and conjugate fetal eye movements, blinking responses, and pupillary reflexes, are reflexes (as there is little to see and as the visual pathways are poorly myelinated). These reflexive behaviors also appear to be associated with high levels of brainstem arousal (e.g., Blessing, 1997; Steriade & McClarley, 1990), as they are reflexively initiated in association with alterations in FHR and body movement including head turning (Martin, 1992; Nijhuis, 1992), and in response to vibroacoustic-startle (Birnholz & Benacerraf, 1983).
THE SLEEP CYCLE AND RAPID EYE MOVEMENTS
Just as high levels of arousal in the newborn are associated with sleep onset and REM (Emde & Koenig, 1969; Emde & Metcalf, 1970), near term fetuses (as young as 36-38 weeks) display high arousal sleep states accompanied by REM (Nijhuis et al., 1982; Romanini & Rizzo, 1995). Neonates and the fetus display similar wake-sleep cycles (Groome, Swiber, Atterbury, Bentz, & Holland, 1997), as well as REM and non-REM periods which are similar to an adult (Emde & Koenig, 1969; Emde & Metcalf, 1970).
There are major differences between the infant/fetal REM sleep cycle and that of adults. Neonates (and presumably the fetus), immediately enter REM and demonstrate high voltage fast activity (deep "paradoxical" sleep), whereas adult sleep onset is characterized by a slow wave, non-REM (light sleep) cycle (Emde & Koenig, 1969; Emde & Metcalf, 1970; Steriade & McCarley, 1990). The adult sleep cycle is then followed by a number of intermediate sleep stages which culminate (every 90 minutes or so) in deep sleep as demonstrated by REM and irregular fast EEG activity (as if the brain were highly aroused: hence, the term "paradoxical sleep"). By contrast, the late term fetus (e.g. Nijhuis et al., 1982), and neonate initially have only two sleep cycles, REM and non-REM (active/quiet sleep). Only around 3-4 months of age does the adult pattern of sleep appear (Emde & Koenig, 1969; Emde & Metcalf, 1970).
Nevertheless, be it fetus, infant, or adult, REM and the control of the sleep cycle are mediated by the brainstem (Steriade & McCarley, 1990), though the adult forebrain exerts some influences on sleep onset. However, the infant forebrain is so immature, and because the brainstem is still maturing, infants will demonstrate REM during sleep as well as during wakefullness. Infants will drift into REM sleep when aroused, with eyes open, and when crying, fussing, eating, or sucking (Emde & Koenig, 1969; Emde & Metcalf, 1970).
It is only as the brain continues to mature and the forebrain increasingly establishes hierarchical control, that the fetal/neonatal sleep cycle is replaced by adult patterns. However, at this later stage of infant/forebrain development, behaviors mediated by the brainstem become increasingly emotionally and cognitively driven, and thus goal directed and purposeful.
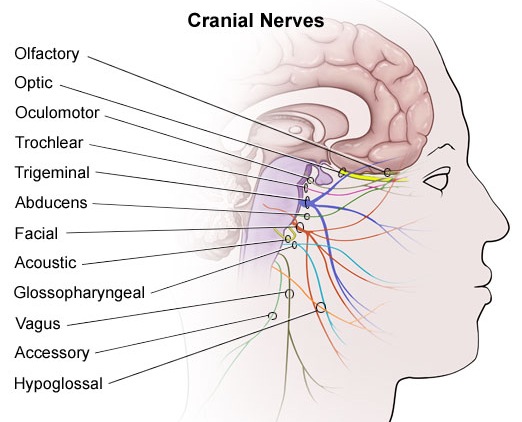
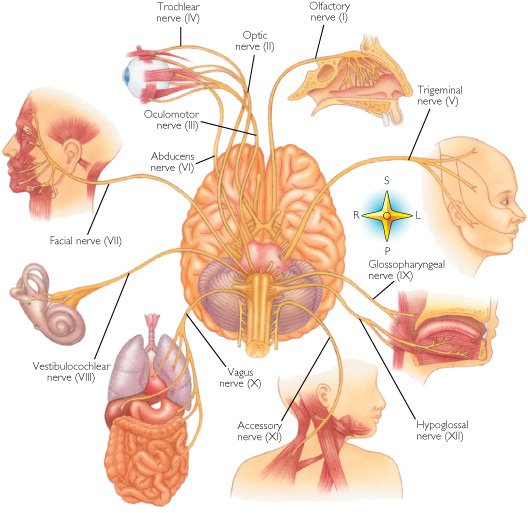
CONCLUSION
It is evident that the fetus is capable of considerable behavioral complexity. These complex actions appear to be mediated and governed by the brainstem with minimal forebrain participation, for similar behaviors are demonstrated by anencephalics and following forebrain destruction. However, although forebrain influences are minimal, the late-term fetal brainstem may also be capable of experienced-induced synaptic plasticity, and can become organized to respond selectively to certain auditory stimuli presented up to 6 weeks before birth. These latter findings could be interpreted as evidence for exceedingly rudimentary, learning-related cognitive-like activity.
Nevertheless, the fetus and neonate appears incapable of thinking, reasoning, understanding, comprehending, or experiencing or generating "true" emotion or any semblance of higher order, forebrain mediated cognitive activity. Rather, although capable of learning, the increasingly complex behaviors demonstrated by the fetus and neonate, including head turning, eye movements, startle reactions, crying, screaming, and rudimentary smiling, are probably best described as brainstem reflexes.