Rhawn Gabriel Joseph, Ph.D.
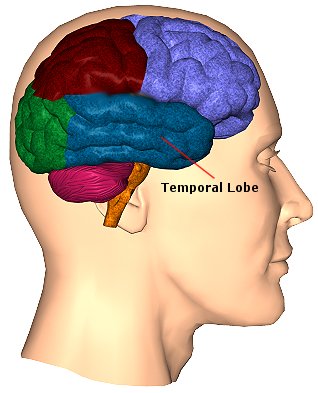
The temporal lobes are unique. These are the only regions of the brain that subserves personalized, subjective emotional and social experience and can store and recall this information from memory. The temporal lobes also contains the core structures of the limbic system involved in emotion and memory, the amygdala and hippocampus.
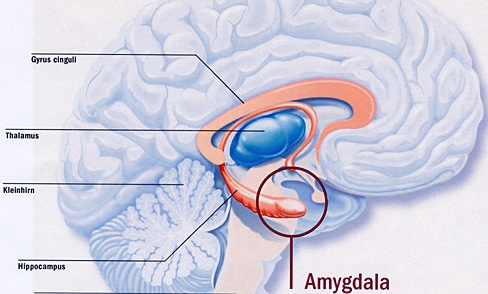
The temporal lobe/limbic allocortex is responsive to complex auditory and visual stimuli (Binder et al., 2014; Gross & Graziano 1995; Nakamura et al. 2014; Nelken et al., 2008; Nishimura et al., 2008; Price, 2011; Rolls 2002; Tovee et al. 2014), comprehending, in humans, complex speech, and contains the highest density and the greatest diversity of peptides and neurotransmitters as compared to all other brain regions (Niewenhujs, 2015).
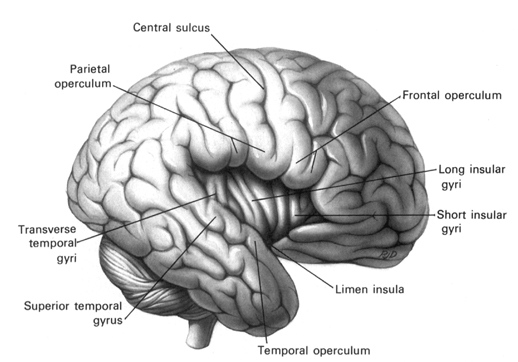
Of all brain regions, only stimulation or activation of the temporal lobe or underlying limbic structures, gives rise to personalized, subjective, emotional, and sexual experiences (Gloor, 2011; Halgren, 2002). Although direct electrical stimulation of the frontal motor cortex can induce twitching of the lips, flexion or extension of a single finger joint, protrusion of the tongue or elevation of the palate, patients never claim to have willed these movements. That is, stimulation of the frontal lobes evokes behavior that seems outside the control of the patient (Penfield & Boldrey, 1937; Penfield & Jasper, 1954; Penfield & Rasmussen, 1950; Rothwell et al. 2007).
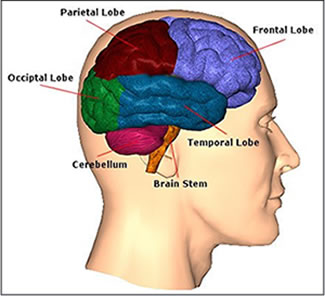
By contrast, electrode stimulation of the temporal lobes evokes experiences which become part of the subjective stream of consciousness, embedded into the very fabric of the personality, such that the personality, and even sexual orientation may be altered. Moreover, patients may experience profound visual and auditory hallucinations and even feel as if they have left their bodies and are floating in space or soaring across the heavens.
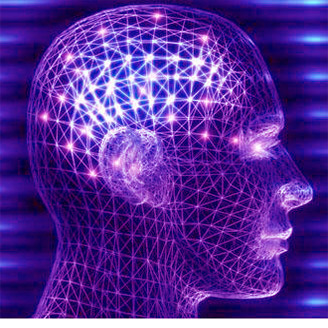
There is no subjective sensations with frontal or parietal or occipital stimulation. In fact, with stimulation to these other brain areas the patient only becomes aware of the stimulation when they attempt to speak or move, or if a finger begins to twitch or if they feel painful tingling or see flashes of light. Although damage to the frontal lobe can produce the "frontal lobe personality" it is the temporal lobe which subserves those aspects of experience which are experienced as personal and subjective, of pertaining to the self and one's personal and even spiritual identity. Indeed, stimulation of the temporal lobe can give rise to profound personal, emotional, sexual, and even religious feelings which are experienced as personally, spiritually and philosophically meaningful, including even sensations of having the "truth" revealed and of receiving knowledge regarding the meaning of life and death.
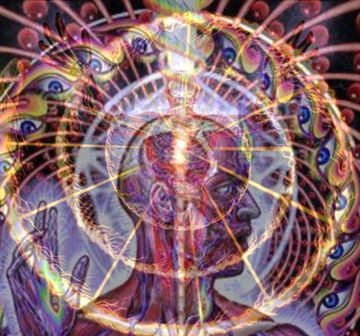
TEMPORAL TOPOGRAPHY
The temporal lobe is the most heterogenous of the four lobes of the human brain, as it consists of six layered neocortex, four to five layered mesocortex, and 3 layered allocortex, with the hippocampus and amygdala forming its limbic core.
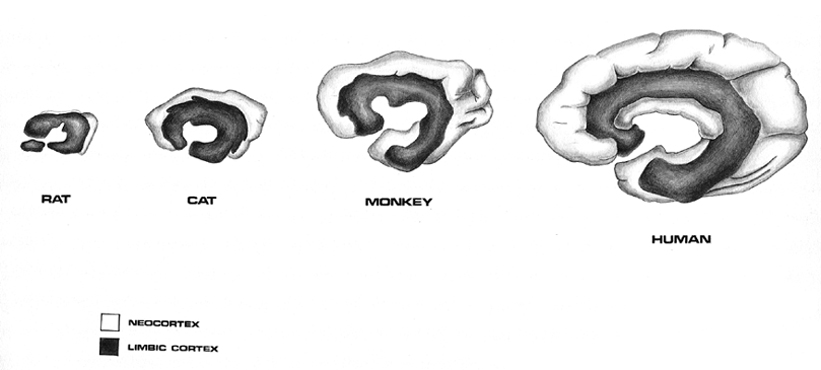
As detailed in chapters, 5, 12, over the course of evolution the dorsally situated hippocampus became displaced and progressively assumed a ventral position, during the course of which it also contributed to the neocortical development of portions of the parietal, occipital and temporal lobe. Similarly, portions of the amygdala became increasingly cortical in structure, and together with the hippocampus, contributed to the evolution of the anterior, medial, superior, and lateral temporal lobes.
Because so much of the temporal lobe evolved from these limbic nuclei, unlike the other lobes, it consists of a mixture of allocortex, mesocortex, and neocortex, with allocortex and mesocortex being especially prominent in and around the medial-anterior inferiorally located uncus, beneath which and which abuts the amygdala and hippocampus.
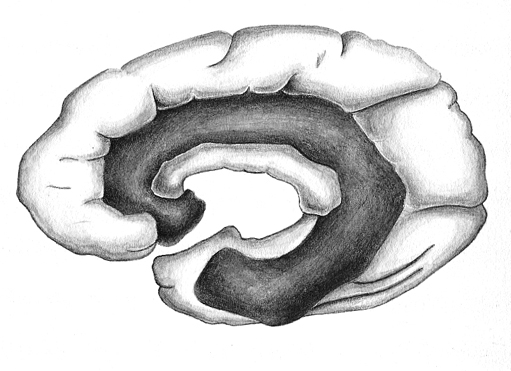
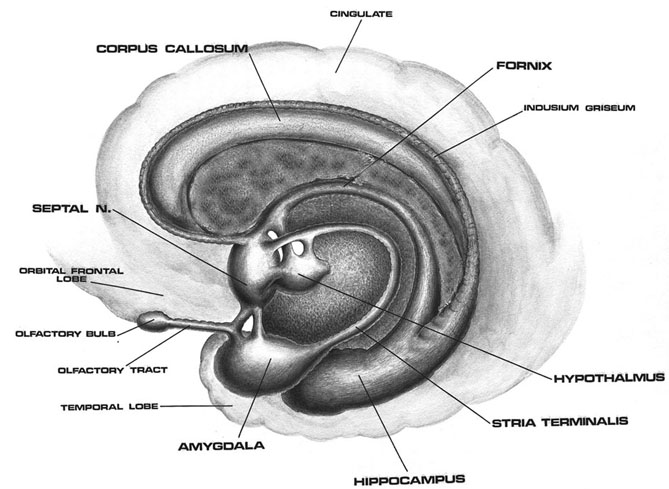
Given the role of the amygdala and hippocampus in memory, emotion, attention, and the processing of complex auditory and visual stimuli, the temporal lobe became similarly organized (Gloor, 2011). Broadly considered, the neocortical surface of the temporal lobes can be subdivided into three main convolutions, the superior, middle, and inferior temporal gyri, which in turn are separated and distinguished by the sylvian fissure and the superior, middle, and inferior temporal sulci. Each of these subdivision performs different (albeit overlapping) functions, i.e. auditory, visual, and auditory-visual-affective perception including memory storage.
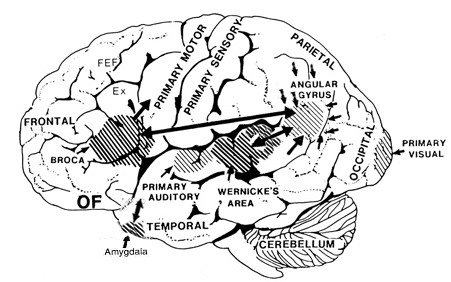
Specifically, the anterior and superior temporal lobes are concerned with complex auditory and linguistic functioning and the comprehension of language (Binder et al., 2014; Edeline et al. 1990; Geschwind 1965; Goodglass & Kaplan, 2008; Keser et al. 2011; Nelken et al., 2008; Nishimura et al., 2008; Price, 2011). The importance of the superior temporal lobe in auditory functioning, and (in the left hemisphere) language, is indicated by the fact that the superior temporal, including the primary auditory area, is larger in humans versus other primates and mammals. In addition, Heschl's gyri, a region of the brain directly implicated in auditory processing, are larger on the left, and the left planum temporal, also an auditory area, is 10 times larger (among the majority of the population) than its counterpart on the right (Geschwind & Levinsky, 1968).
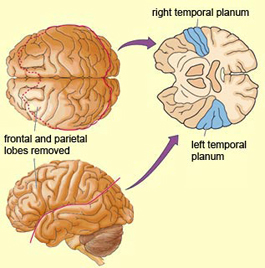
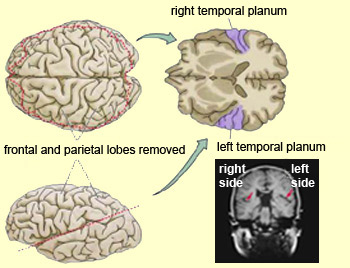
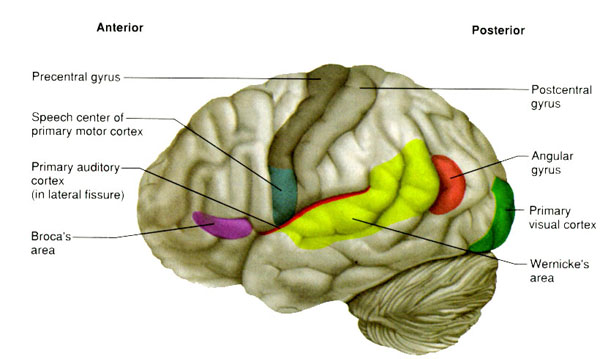
The auditory areas, however, extend along the axis of the superior temporal lobe, extending in a anterior-inferior and posterior-superior arc, such that the connections of the primary auditory area extends outward in all directions throughout the brain, innervating, in a temporal sequences, the second and third order auditory association areas (Pandya & Yeterian, 2015). Thus, each area of the brain involved in auditory processing, from simple to complex analysis, are interlinked and these association areas in turn project back to the primary auditory area.
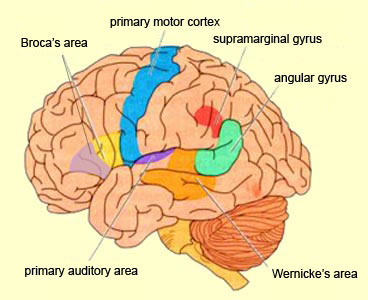

Vision and the Temporal Lobes
The temporal lobes perform complex analysis of visual stimuli, including the recognition of faces, animals, tools, houses, and other complex geometric forms.
The inferior and medial temporal lobe harbors the amygdala and hippocampus, performs complex visual integrative activities including visual closure, and contains neurons which respond selectively to faces and to the sight of complex objects (Gross & Graziano 1995; Nakamura et al. 2014; Rolls 2002; Tovee et al. 2014).
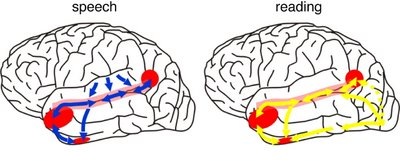
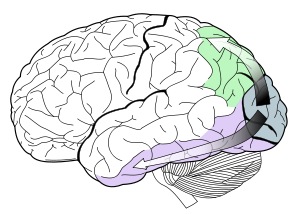

The inferior and middle temporal lobes, are the recipients of one two diverging (dorsal and ventral) streams of visual input arising from within the occipital lobe and thalamus (Ungerlieder & Mishkin, 1982); i.e. the pulvinar and dorsal medial nucleus of the thalamus. The dorsal stream is more concerned with the detection of motion and movement, orientation, binocular disparity, whereas the ventral stream is concerned with the discrimination of shapes, textures, objects and faces, including individual faces (Baylis et al., 2015; Perrett et al., 2004, 2002). This information flows from the primary visual to visual association areas and is received and processed in the temporal lobes and is then shunted to parietal lobe, and to the amygdala and entorhinal cortex (the gateway to the hippocampus) where it may then be learned and stored in memory.
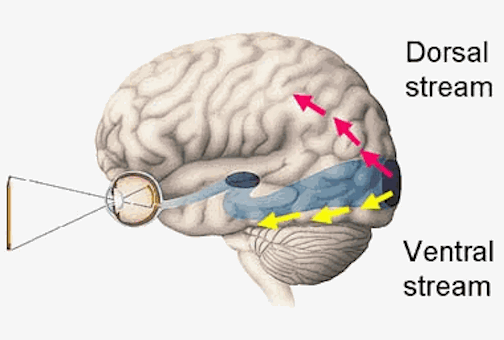
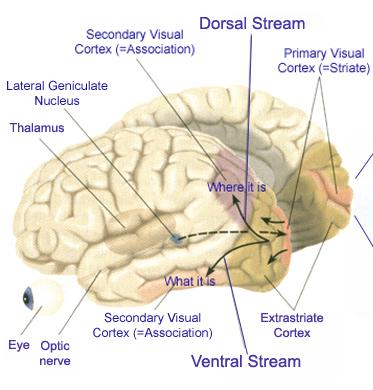
The temporal lobes, however, also receive extensive projections from the somesthetic and visual association areas, and processes gustatory, visceral, and olfactory sensations (Jones & Powell, 1970; Previc 1990; Seltzer & Pandya, 1978) including the feeling of hunger (Fisher 2014). Hence, the temporal lobes perform an exceedingly complex array of divergent and interrelated functions.
FUNCTIONAL OVERVIEW
The right and left temporal lobe are functionally lateralized, with the left being more concerned with non-emotional language functions, including, via the inferior-medial and basal temporal lobes reading and verbal (as verbal-visual) memory. For example, as determined based on functional imaging, when reading and speaking the left posterior temporal lobe becomes highly active, due, presumably to its involvement in lexical processing (Binder et al., 2014; Howard et al., 199). The superior temporal lobe (and supramarginal gyrus) also becomes more active when reading aloud than when reading silently (Bookheimer, et al., 1995), and becomes active during semantic processing as does the left angular gyrus (Price, 2011).
These same temporal areas are activated during word generation (Shaywitz, et al., 1995; Warburton, et al., 1996), and sentence comprehension tasks (Bottini, et al., 2014; Fletcher et al., 1995). and (in conjunction with the angular gyrus) becomes highly active when retrieving the meaning of words during semantic processing and semantic decision tasks (Price, 2011). Likewise, single cell recordings from the auditory areas in the temporal lobe demonstrate that neurons become activated in response to speech, including the sound of the patient's own voice (Creutzfeldt, et al., 2008).
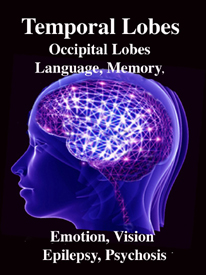
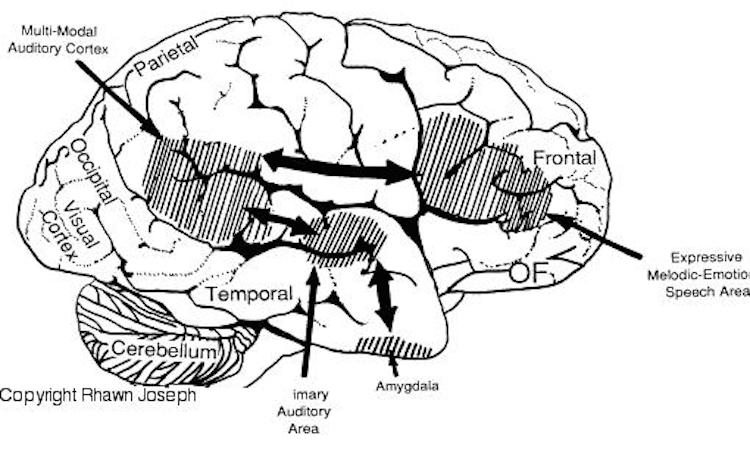
By contrast, the right is more concerned with perceiving emotional and melodic auditory signals (Evers et al., 2008; Parsons & Fox, 2011; Ross, 1993), and is dominant for storing and recalling emotional and visual memories (Abrams & Taylor, 1979; Cimino, et al. 2011; Cohen, Penick & Tarter, 1974; Deglin & Nikolaenko, 1975; Kimura, 1963; Shagass et al.,1979; Wexler, 1973). The right temporal lobe becomes highly active when engage in interpreting the figurative aspects of language (Bottini et al., 2014).
However, there is considerable functional overlap and these structures often become simultaneously activated when performing various tasks. For example, as based on functional imaging, when reading, the right posterior temporal cortex also becomes highly active (Bookheimer, et al., 1995; Bottini et al., 2014; Price, et al., 1996), and when making semantic decisions (involving reading words with similar meanings), there is increased activity bilaterally (Shaywitz, et al., 1995).
Presumably, in part, both temporal lobes become activated when speaking and reading, due to the left temporal lobe's specialization for extracting the semantic, temporal, sequential, and the syntactic elements of speech, thereby making language comprehension possible. By contrast, the right temporal lobe becomes active as it attempts to perceive, extract and comprehend the emotional (as well as the semantic) and gestalt aspects of speech and written language.
THE INFERIOR & MIDDLE TEMPORAL LOBE
The basal temporal region can be subdivided into a medial, inferior, middle, and anterior and posterior zones, harbors the amygdala and hippocampus in its depths. These tissues are extensively interconnected, and project to the auditory association area, frontal convexity, and orbital region (Jones & Powell, 1970; Pandya & Kuypers, 1969). The inferior arcuate fasciculus in it's journey from the amygdala to Wernickes areas also passes through this neocortical auditory territory. Hence, when considered a whole, the inferior and middle temporal lobe is a complex multifunctional tissue which is functionally lateralized and has extensive visual and auditory and linguistic capabilities.
In general, the anterior basal temporal lobe is more involved in auditory functioning, whereas the posterior basal temporal lobe is more intimately concerned with the processing of higher level visual information including form recognition and (in the left temporal lobe) the reading of the written language. Indeed, this generalized dichotomy, first proposed in the 1990 edition of this text, has since been born out by a variety of functional imaging studies and is supported by lesions and neurosurgical stimulation studies.
Specifically, the left inferior-middle posterior temporal lobe (Brodmann's area 37), is located between the visual cortex and the anterior temporal cortex and becomes activated during a variety of language tasks, including reading and object and letter naming (Price, 2011). This has been demonstrated not only by functional imaging (Buchel et al., 1998; Price, 2011), but direct cortical recoding (Nobre et al., 2014), and electrical stimulation (Luders et al., 1986). In fact, both normal, cognitally blind, and late-blind subject display activity in this area (Buchel et al., 1998). For example, reading of concrete and abstract words activates the left posterior basal temporal lobe and the left frontal operculum (Buchel et al., 1998).
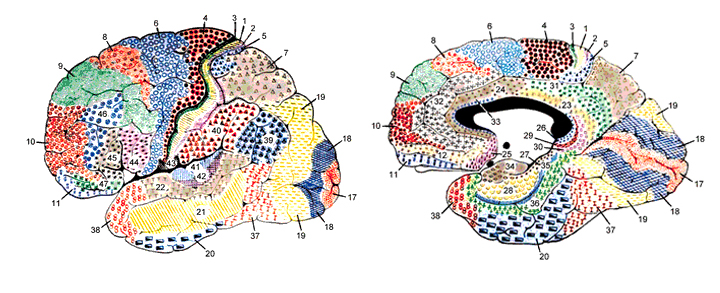
Hence, because of its role in language, when the middle temporal lobe is stimulated there can result aphasic abnormalities (Penfield & Rasmussen, 1950). Lesions involving this area are also associated with subtle disturbances involving language, including word finding difficulty, confrontive naming deficits, abnormalities in the maintenance of temporal order and sequence, as well as verbal memory impairments (Luria, 1980). Patients may suffer from reading and naming deficits (Rapcsak, et al., 2007); a condition referred to as phonological alexia. Moreover, patients with developmental dyslexia have been found to have abnormalities in this area (Rumsey et al., 2011).
As noted, left temporal lobe activity increases as word length increases. Hence, with injuries in this area, including the basal temporal lobe, patients may have difficulty recalling word lists or order of word presentation, such that the longer the list, the greater the difficulty. Hence, if a list of four words were read, the patient cannot repeat the correct order even after repeated presentations, although individuals words may be recalled correctly (Luria, 1980).
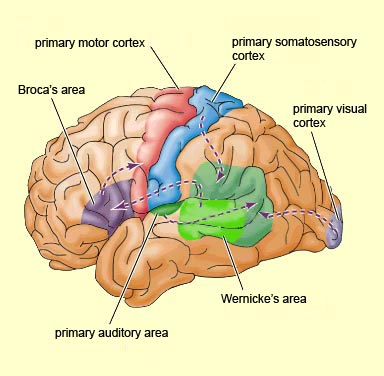
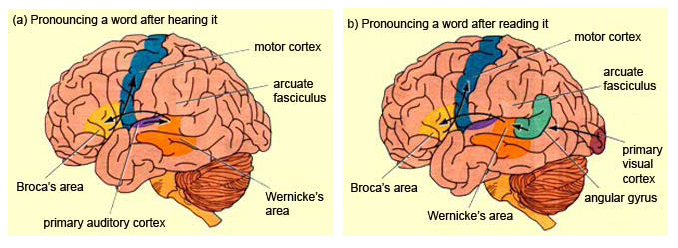
However, the nature of the disturbances depends on the exact location of the lesion (Coltheart, 1998; Miozzo & Caramazza, 1998), for as noted, the anterior and posterior and inferior and middle temporal lobes perform interrelated but also divergent functions. In general, the more posterior aspect is concerned with the visual aspects of perception and language, including reading and object naming. Indeed, the left posterior basal temporal lobe is a convergence zone for visual, auditory, and tactile information. Hence, patients may suffer from alexia as well as visual and tactile anomia if this area is injured (Rapcsak, et al., 2007). In addition, these structures are lateralized, and although both the right and left temporal lobe become activated by various language and reading tasks (reviewed in Price, 2011), the right basal temporal lobe is much more concerned with non-verbal visual functioning.
THE 'VISUAL" MIDDLE INFERIOR TEMPORAL LOBE
The middle temporal lobe, (MTL) like other structures throughout the brain, appears to be functionaly lateralized such that the right temporal region is more involved in visual and non-verbal auditory functioning, whereas the left MTL is more concerned with auditory-linguistic capabilities. Hence, whereas the auditory-linguistic zones appear to be more extensive within the left temporal lobe (and include portions of the posterior temporal region), the right MTL seems to be more associated with visual responsiveness. In general, however, the middle temporal gyrus of both hemispheres appear to contain visually responsive neurons (Beckers & Zeki 1995; Felleman & Kaas, 1974; Heit et al. 1990; Maunsell & Van Essen 2004; Selemon et al. 2014). Nevertheless, since there have been relatively few published studies of the effects of lesions or the functional capabilities of the middle temporal lobes in humans, notions regarding the extensiveness of auditory vs. visual lateralized representation are admittedly speculative.
MIDDLE AND INFERIOR TEMPORAL LOBE VISUAL FUNCTIONING
Visual cells in the MTL receive direct projections from the striate cortex, area 17 and the association area 18 (Wall et al. 1982). This area in turns projects to the MTL of the opposite hemisphere via the corpus callosum, ipsilaterally to areas 17, 18, 19, the inferior temporal and the parietal-occipital cortex (Tigges et al., 2011; Wall et al., 1982), and maintains interconnections with the pulvinar, superior colliculus and pontine nuclei of the brainstem (Walls et al., 1982). Hence, this regions has connections with areas concerned with visual and visual attentional capabilities.

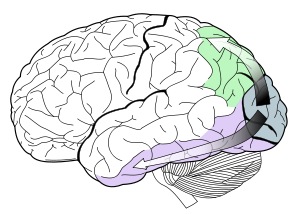
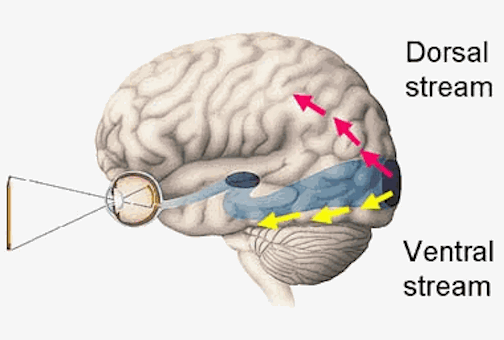
MTL neurons are sensitive to speed and direction of stimulus movement, motion, orientation, width, and disparity (Felleman & Kaas, 2004; Maunsell & Van Essen, 1983). However, most MTG neurons are not particular sensitive to the form of a stimulus--most preferring narrow stimuli (Albright et al. 2004; Maunsell & Van Essen, 1983). A large number of cells have binocular capabilities and respond to stimuli from either or both eyes. Hence, these cells are significantly involved in stereoscopic vision as well as determining distance.
Although not specialized for perception of form per se, via the combined analysis of width, orientation, speed and direction of movement these cells can process visual stimuli in a three-dimensional fashion, particularly in regard to trajectory, depth and position, i.e. if an object is near or far, approaching or withdrawing (Maunsell & Van essen, 1983).
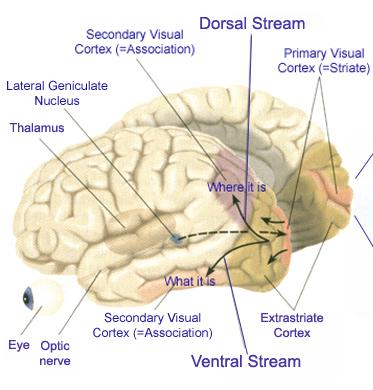
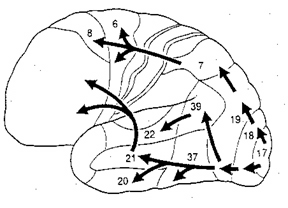
Via its interconnections with the parietal-occipital lobe (an area involved in depth perception, visual fixation, and the visual-somesthetic guidance of movement) the MTL appears to provide visual guidance in regard to interactional object and body movement. That is, via the analysis of the flow of movement, local direction and speed, as well as the relative distances of objects, these neurons (in conjunction with area 7 cells) are important in guiding the movements of the body as well as the individuals limbs toward the object (Manunsell & Van Essen,1983).
The MTL maintains extensive interconnections with the inferior region and thus with limbic nuclei. It is probably via these connections that objects or conspecies of motivational significance can be discerned and attended to. That is, not only is the speed, movement, distance, etc. of a stimulus determined, but its emotional attributes (e.g. should it be feared, chased, eaten). Moreover, not just written words, but object recognition occurs in this area (in conjunction with the inferior temporal lobe), such that if injured, patients may suffer from agnosia, i.e. an inability to recognize visual images (Giannokapoulos et al., 2008).
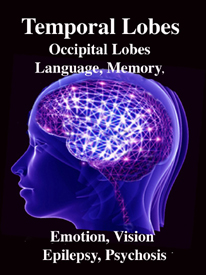
HALLUCINATIONS.
Tumors involving the middle temporal lobe have been associated with the development of auditory and visual hallucinations, dreamy states, and alterations in emotional functioning--particularly as the lesion encroaches on the inferior regions (Luria, 1980). Electrical stimulation also initiates the development of complex hallucinations and alterations in consciousness (Penfield & Roberts, 1959).
INFERIOR TEMPORAL LOBE
As noted, it is possible to very loosely define the anterior-inferior temporal lobes as auditory cortex, although neurons in this vicinity receive visual as well as somesthetic input.. Hence, the inferior temporal lobes can respond to auditory as well as emotionally and visually significant stimuli (Gloor, 2011; Nakamura et al., 2014; Tovee et al., 2014).
That the inferior temporal lobe (ITL) processes complex auditory, visual and emotional stimuli is probably a function of it being a derivative of the amygdala (see chapter 14) as well as visual cortex (Diamond 1973; Gloor, 2011). Visual and auditory functions also reflect its reception of extensive (upper visual field) projections from the optic radiations, highly processed input from the visual association areas in the occipital lobes of both cerebral hemispheres (via the corpus callosum), and fibers from the superior colliculus by way of the pulvinar of the thalamus (Chow, 1950; Kaas & Krubitzer 2011; Kuypers et al., 1965; Previc 1990; Rocha-Miranda et al. 1975).
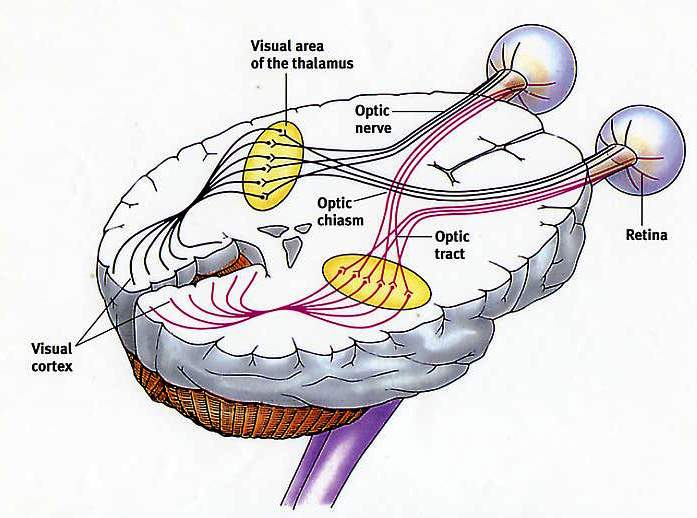
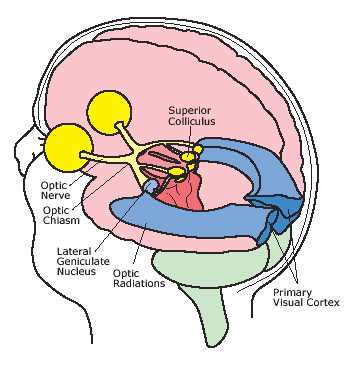
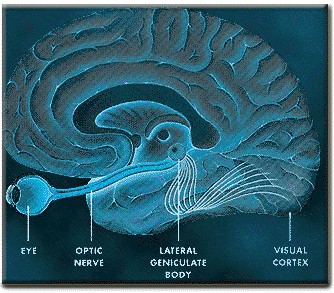
VISUAL CAPABILITIES & FORM RECOGNITION
Indeed, the neocortex of the ITL is specialized for receiving, analyzing, discriminating, recognizing and recalling complex visual information and is involved in attention and visually guided behavior (Gross & Graziano 1995; Gross et al. 1972; Tovee et al. 2014), including the recollection and learning of visual discriminations (Gross, 1972) and memory for objects and spatial locations (Nunn et al., 2008; Ploner et al., 2008). If the temporal lobe is injured, these functions become compromised.
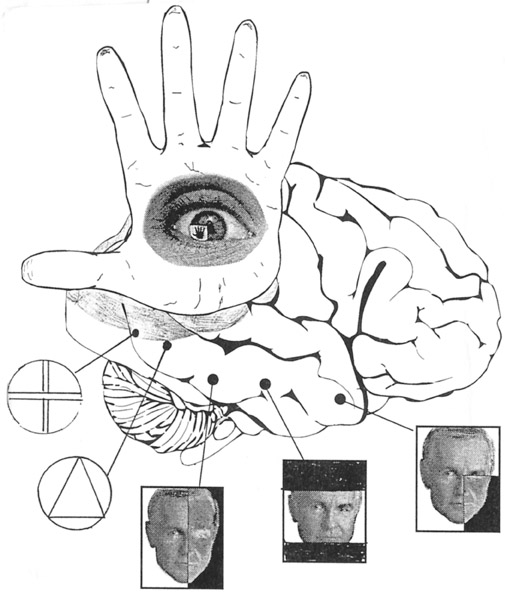
For example, Kimura (1963) found that patients with right vs left temporal lobe injury were impaired when presented with overlapping nonsense shapes and then immediately tested for recognition. Likewise, Meier and French (1965), found that those with right vs left temporal lobe injuries were impaired when asked to make visual discriminations when presented with fragmented concentric circle patterns--skills which are also related to visual closure and gestalt formation. More recently, Nunn et al., (2008) found that right vs left temporal lobectomy patients were more impaired when required to remember and recognize toys and recall their location.
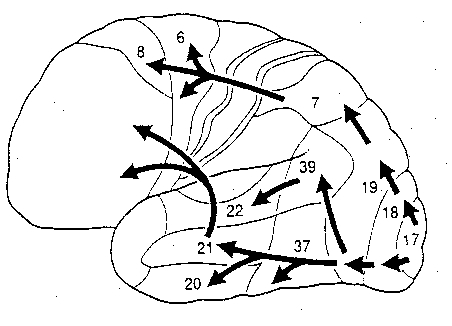
Cells in the ITL have very large, bilateral visual receptive fields which include the fovea, and many are sensitive to direction of stimulus movement, color, contrast, size, shape, orientation and are involved in the perception of three dimensional objects and the supramodal analysis of information already processed in the association areas (Eskander, et al. 2002; Gross & Graziano 1995; Gross, et al. 1972; Nakamura et al. 2014; Rolls, 2002; Sergent, et al.1990). Thus inferior temporal (IT) neurons appear to take part in the last stages of visual analysis for form recognition and receive terminal fibers from the primary and association visual areas (Gloor, 2011; Gross, et al. 1972; Ungerleider & Mishkin, 1982); that is, via the ventral visual stream. Indeed, a single neuron can respond to a combination of these features and many will fire selectively in response to particular shapes and faces (Eskander, et al. 2002; Gross, et al. 1972; Nakamura et al. 2014; Richmond, et al. 1983; Rolls, 2002; Sergent, et al.1990).
FORM & FACIAL RECOGNITION
Overall, the ITL appears to be involved in the highest level of visual integration containing highly developed neurons which seem to be the end station of a hierarchical system which mediates the perception and recognition of specific and particular shapes and forms (Desimone & Schein, 2007; Gross & Graziano 1995; Nakamura et al. 2014; Richmond, et al. 1983; Rolls, 2002.) Indeed, in addition to the optic radiations, there is a pathway (the ventral stream) beginning in the primary visual cortex which passes through areas 18 and 19 and terminates in the ITL (Kuypers et al., 1965; Previc 1990). As information is passed from the primary to these association areas various features important in the identification of specific objects become progressively and hiarchically analyzed and increasingly complex associations are formed.
In fact, based on single cell recordings, some of these ITL neurons have been found to become particularly excited when presented with two dimensional patterns or three dimensional objects such as hands, brushes, and in particular, faces (Deismone & Gross, 1979; Gross et al., 1972; Nakamura et al. 2014; Richmond et al., 1983; Richmond et al.2007). A variety of different feature detectors are found and the majority probably act collectively so as code and assemble a particular shape, including the formation of gestalts and thus the performance of visual closure, particularly the right temporal region (see chapter 10). Via closure an individual can detect a partial stimulus and recognize that it is a face vs a rabbit.
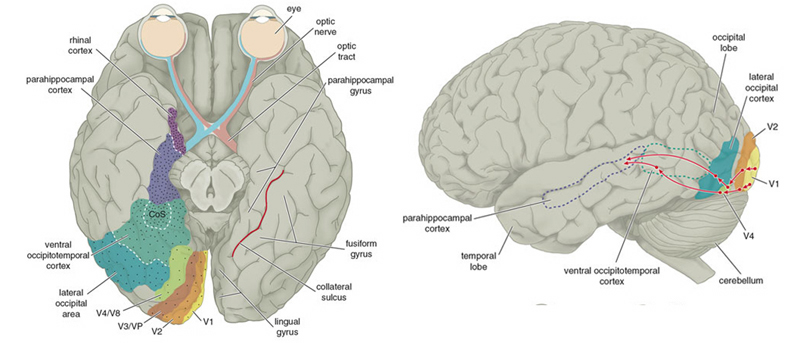
Some cells are responsive to particular facial orientations, such as a profile, some respond to only parts of the face, such as eyes or a mouth, whereas others respond only to the entire face; i.e. a correctly organized facial gestalt. Interestingly, the ITL also contains neurons which will fire even to a scrambled face, so long as all the features are present (Gross et al., 2004). Moreover, some cells respond preferentially to familiar faces, to the faces of certain individuals, to specific emotions conveyed via the face, and the direction of the face including the direction of gaze--especially cells within the amygdala. Specifically, the left amygdala acts to discriminate the direction of another person's gaze, whereas the right amygdala becomes activated while making eye-to-eye contact (Kawashima, et al., 2008). Hence, these cells can determine if someone is looking at them, or looking at someone else, if the facial expression conveys threat, and if there is a hand moving toward or away from the subject or another person.
Hence, these cells are especially equipped for determining social signals and to read the emotions conveyed facially between two people. Interestingly, these same capacities were originally mediated by the olfactory system which in turn gave rise to the amygdala and promoted its differentiation and thus the development of the temporal lobe. In this regard it is also noteworthy that with the bilateral removal of the anterior inferior temporal lobe (thus destroying the amygdala as well as parts of the hippocampus), patients (and non-human primates) develop so called "psychic blindness," and will pick up and smell and taste whatever object captures their attention. In other words, being deprived of an amygdala that can process, visually, social and emotional signals, the amygdalectomized patient/primate reverts back to those behaviors typical of creatures that rely on smell.
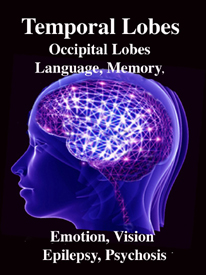
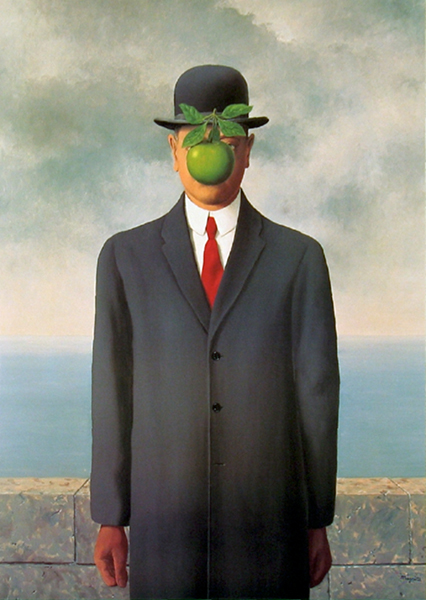
AGNOSIA AND TEMPORAL LOBE FUNCTIONAL LATERALITY
As noted, abnormalities affecting the middle temporal lobe (area 37) can result in agnosic disturbances (Giannokapoulos et al., 2008). There are however, to major forms of agnosia, and different subtypes as well, which can be produced by damage to tissues mediating complex visual perception or lesions disconnecting the IPL, for example, from visual, somesthetic, or auditory input.
For example, one type of agnosia, "apperceptive visual agnosia" refers to a disturbance in perceptual and visual-motor integration, such that patients have difficulty copying or matching various objects. This latter form of agnosia has been associated with lesions to the parietal occipital cortex (Mizuno, et al., 1996), as well as to bilateral damage to the inferior-occipital cortex (Shelton, et al., 2014).
By contrast, associative visual agnosia, which is a deficit in naming, such that auditory equivalents cannot be matched to a visual perception, is associated with left inferior and middle temporal (area 37) occipital abnormalities (Giannokapoulos et al., 2008), which may be accompanied with alexia (Feinberg et al., 2014), as well as to lesions to and atrophy of the parietal occipital cortex (Mizuno, et al., 1996).
It is noteworthy that depending on the laterality and location of the injury, e.g., right vs left inferior/medial vs superior temporal lobe, patients may display category specific agnosias. For example, a 27-year old man I examined who had sustained a massive right inferior-posterior temporal lobe injury that also involved surgical removal, was able to recognize pictures of tools (he had been a carpenter) but could not recognize or correctly name pictures of animals and he could not correctly remember facial stimuli that he had been shown five minutes earlier and could not differentiate them from faces he had not seen. By contrast, a 43 year old woman who had been a waitress, and had developed a left inferior temporal lobe glioma that required surgical removal (coupled with chemotherapy) was able to recognize and name pictures of animals and could remember different pictures of faces, but had considerable difficulty recognizing and naming common household objects.
In this regard a 47-year old woman I examined who was subsequently found to have a calcium cyst growing from the skull into the right superior temporal lobe, was able to name pictures of animals, tools and household objects. However, she was almost completely unable to recognize and correctly name animal and humans sounds (e.g. a baby crying, a crowd cheering, a lion roaring) which had been briefly presented, but was better able to recognize non-living sounds such as a creaking door, or a hammer hammering--though these abilities were also compromised.
These findings, which require independent confirmation, raises the possibility that the temporal lobes are not only able to distinguish between living and non-living things including tools and faces, but that the right temporal lobe is specialized for perceiving living creatures (and the sounds they make) whereas the left is specialized for perceiving and naming non-living things, such as tools and household objects.
VISUAL ATTENTION
Attention and visual fixation involves the activation of neurons in the superior parietal and frontal eye fields, the midbrain colliculi, and thalamic nuclei--regions with which the ITL maintains interconnections (Gloor, 2011; Gross & Graziano 1995; Previc 1990). Attention, however, generally requires that visual fixation be focused. Since many ITL neurons maintain large bilateral visual fields, when engaged in visual fixation ITL these cells appear to become partly suppressed. That is, the area of the visual field they respond to becomes restricted to the fovea (Pevic 1990; Richmond et al., 1983). In this manner, ITL neurons become less responsive to other objects such as those in the periphery as the receptive field contracts around the object attended to.
Hence, ITL neurons seems to scan the entire visual field so as to alert the organism to objects of interest or motivational importance (via interconnections with limbic nuclei). When detected, the frontal eye fields, the superior parietal lobe, and subcortical neurons are activated triggering visual fixation. Simultaneously, ITL visual form recognition neurons are activated whereas those with wide non-specific visual fields are inhibited. In this manner, objects of interest are detected and fixated upon (e.g. Previc 1990).
Of adjunctive importance is the middle temporal lobe which in turn can analyze the velocity and direction of the objects movement so that the individual may approach, and, via interaction with cells in area 7, grasp and manipulate the object.
As based on functional imaging (Sergent, et al., 2002), the medial temporal lobe, the right parahippocampal gyrus (in conjunction with areas 19, 37, 36), and the inferior and middle temporal lobe becomes highly active when viewing and categorizing faces and other complex stimuli. Likwise, electrical stimulation of these areas can produce hallucinations and memories of faces of complex visual stimuli (Gloor, 2011; Halgren, 2002).
Damage or removal of the inferior temporal lobe results in loss of the ability to recognize faces, as well as creating severe disturbances involving visual discrimination learning and retention (Braun et al. 2014; Gross & Mishkin, 1977; Mishkin, 1972), and difficulty performing visual closure and recognizing incomplete figural stimuli (Kimura, 1963; Lansdell, 1968,1970). For example, primates with lesions in this vicinity, have severe difficulty learning to discriminate between different shapes and patterns and objects which differ in regard to size or color, although visual acuity is normal.
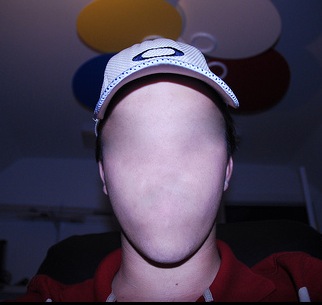
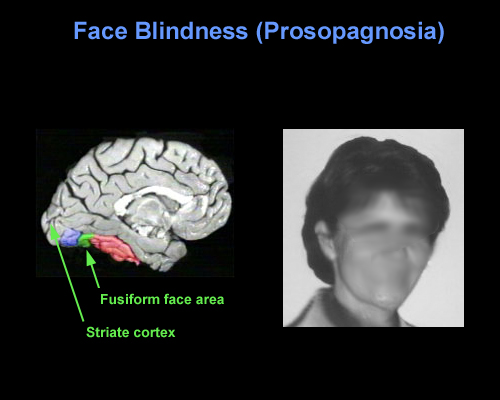
With damage to the right temporal-occipital region, there can result a severe disturbance in the ability to recognize the faces of friends, loved ones, or pets (Braun et al. 2014; DeRenzi, 1986; DeRenzi et. al., 1968; DeRenzi & Spinnler, 1966; Hanley et al. 1990; Hecaen & Angelergues, 1962; Landis et al., 1986; Levine, 1978; Whitely & Warrington, 1977l ) or to discriminate and identify even facial affect (Braun et al. 2014); a condition referred to as prosopagnosia. In fact, with gradual deterioration and degeneration of the right inferior temporal lobe, patients may suffer a progressive prosopagnosia (Evans et al. 1995). Some patients may in fact be unable to recognize their own face in the mirror. For example, one patient was unable to even discriminate between people on the basis of sex but instead had to relie on the presence of details, such as lipstick, rouge, hair length, a moustache, so as to make discriminations (Levine, 1978).
Presumably, the inability to recognize faces is due to the destruction of facial recognition neurons, including those within the amygdala (Young et al. 1995) and hippocampus (which becomes activated when memorizing faces, Kapur et al. 1995). However, because, at a neocortical level, global facial recognition cells appear to be in a minority whereas those specialized for analyzing facial parts are more numerous, the ability to recognize facial details is therefore more likely to be preserved following inferior temporal destruction -particularly if the left temporal lobe is spared.
Nevertheless, the association with the amygdala is particularly important, as facial stimuli are also social emotional stimuli, and facial recognition, for example, that of a friend or loved one, is generally associated with emotional feelings. Moreover it is via the face that emotional intent and affective states, including threat or fear, is conveyed--information that is processed by the amygdala (e.g. Morris et al., 1996). Hence, damage to the overlying temporal lobe may result in prosopagnosia, including an inability to recognize the face of friends or relatives, due to also to disconnection and an inability to attribute and associate the emotion of recognition to these facial stimuli. It is perhaps for this reason, that some patients with prosopagnosia are also unable to recognize their pets or their homes--the emotional significance of these stimuli can no longer be evoked. These stimuli are no longer personalized and thus cannot be recognized.
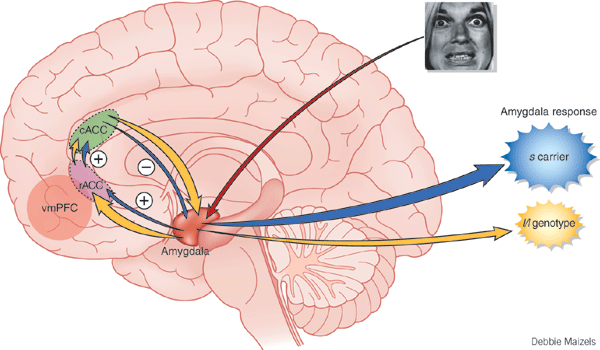
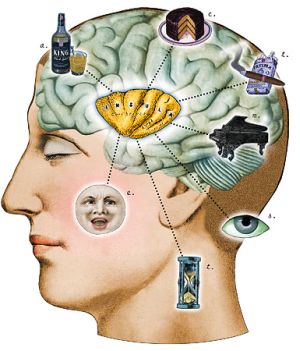
Lateralization & Facial Recognition.
Although patients with prosopagnosia often suffer from bilateral injuries, in many cases the lesions are restricted to the right hemisphere (DeRenzi, 1986; DeRenzi et. al., 1968; DeRenzi & Spinnler, 1966; Evans et al. 1995; Hanley et al. 1990; Hecaen & Angelergues, 1962; Landis et al., 1986; Levine, 1978; Whitely & Warrington, 197l). Disturbances involving facial recognition do not usually occur with isolated left cerebral lesions. Indeed, the right hemisphere appears to be dominant in regard to the recognition of both familiar and unfamiliar faces as has been well demonstrated in numerous studies of brain injured as well as normal, intact individuals (Bradshaw et al. 1980; DeRenzi, 1982; DeRenzi et al.1968; DeRenzi & Spinnler, 1966; Evans et al. 1995; Geffen et al. 1971; Levy et al. 1972; Ley & Bryden, 1977; Hecaen & Angelergues, 1962). The left hemisphere, however, is involved in the recognition of famous faces (Marzi & Berlucchi, 1977; Rizzolatti, et al. 1971; Hanley et al. 1990).
Among neurosurgical patients, it has also been reported that electrical stimulation of the posterior right temporal gyrus disrupts visual-spatial memory for faces in general (Fried et al., 1982). Electrical stimulation of the posterior portion of the right middle temporal gyrus also results in an inability to correctly lable emotion faces.
Agnosias.
When the ITL is damaged, in addition to prosopagnosia there may occur difficulty identifying various familiar stimuli and objects, e.g. utensils, cars, as well as differentiating among similar visual stimuli. Many patients also have difficulty with color recognition (Green & Lessell, 1977; Meadows, 1974b). Frequently these types of agnosic disturbances are related to left cerebral or bilateral dysfunction.
MEMORY
The IT is able to code for and learn new stimuli, can recall the code of the previous stimuli, and can make comparisons between what was perceived on one occasion versus another (Eskandar et al. 2002). This enables IT neurons to make judgments regarding temporal order; if the stimulus was first or second or last (Baylis, & Rolls, 2007; Eskander, et al. 2002). Thus, IT neurons can perform sequential analysis and can compare initial with previous visual representations (Eskandar et al. 2002). Hence, neurons in the IT are involved in form and facial recognition, discrimination, temporal-sequencing, and thus learning and memory for a variety of stimuli.
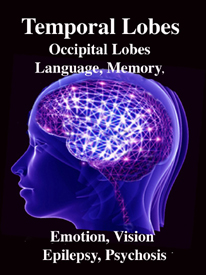
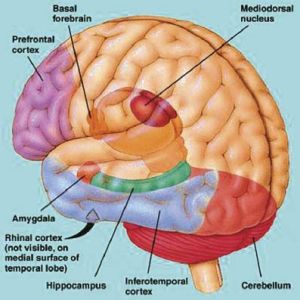
In addition, IT neurons are directly connected with the entorhinal cortex (Jones & Powel, 1970; Van Hoesen & Pandya, 1975b) -the "gateway to the hippocampus"- as well as to the amygdala (Amaral et al. 2007). Thus neurons located in the inferior temporal lobe are also involved in the maintenance of short term emotional, visual and cognitive memory, and many display heightened activity during delay periods while learning (Fuster & Jevey, 2011; Miyashita & Chang, 1988). Moreover, IT neurons are are also highly concerned with the behavioral context in which learning occurs (Baylis & Rolls, 2007; Gross, et al. 1979; Riches, et al. 2011).
For example, Eskandar, et al. (2002) found in their studies of electrophysiological activity that neurons in the inferior temporal gyrus convey information concerning current versus previous stimulus patterns and their behavioral context. IT neurons are therefore involved in remembering this information, matching it with previously learned information, and are capable of simultaneously transmitting these impressions (including contextual details) to other brain areas. However, IT neurons are also capable of discriminating between stimuli independent of context (Eskandar, et al., 2002).
Hence, IT neurons are involved in both encoding, storage, and recall and interact with the amygdala and hippocampus in regard to learning, memory and recognition. It is in this manner and through these interconnects that IT neurons are involved in emotional as well as non-emotional cognitive processing and memory storage. Conversely, with injuries to the inferior temporal lobe, visual and verbal memory functions suffer (see chapter 14).
MEMORY, THE HIPPOCAMPUS & INFERIOR MEDIAL TEMPORAL LOBE
The entorhinal, perirhinal, and parahippocampal neocortex are located in the inferior and medial temporal lobe and are adjacent to and richly interconnected with the hippocampus which is buried within its depths. Like the hippocampus and amygdala, the inferior and medial temporal lobes are involved in memory functioning (Gloor, 1990, 2011; Murray, 2002; Nunn et al., 2008; Ploner et al., 2008; Squire, 2002) and may in fact serve as neuronal memory depots that becomes activated during recognition (e.g. Brewer et al., 1998, Wagner et al., 1998).
As argued by Heit and colleagues (1990), "MTL firing patterns may contribute to the reactivation of neocortical circuits encoding a particular stimulus-context gestalt" which in turn makes recognition and retrieval possible. For example, the medial temporal lobe (MTL) becomes activated during recognition memory tasks including those involving words and faces (Heit et al. 1990). Indeed, the greater the activation, the greater is the likelihood that material will be remembered (e.g. Brewer et al., 1998; Wagner et al., 1998).
When the inferior and medial temporal lobe is electrically stimulated exceedingly vivid personal memories may be triggered and recalled (Gloor, 1990, 2002, 2011; Halgren 2002; Halgren, et al. 1978; Penfield, 1954; Penfield & Perot, 1963). Patients may report seeing a complex scene from their past or early childhood, including hearing conversations, seeing faces, experiencing somesthetic sensations, and related events. Curiously, however, these scenes do not move forward in time and are otherwise quite static (See Gloor, 1990, 2011; Halgren 2002), like a wide angle, multi-sensory snapshot.
Conversely, left MTL lesions results in recognition deficits for words (Milner & Teuber 1968), whereas those involving the right MTL disrupt memory for visual forms and faces (e.g. Hanley et al. 1990). However, the entorhinal cortex and hippocampus appear to be the crucial structures, whereas the neocortex may be the site where memories are stored. Consider, for example, patients who undergo "hippocampal removals" whereas the overlying neocortex is spared (Milner, 1990) and patients such as the famous H.M., who underwent bilateral mesial temporal removals: amygdala, hippocampus, entorhinal cortex (Milner, 1968). These patients (particularly those with right sided destruction) perform exceedingly poorly on visual recognition memory tests, including those involving recurring nonsense figures (Kimura, 1963) and human faces (Milner, 1990).
In that the inferior and inferior medial temporal lobes also contain neurons which are selectively sensitive to particular sensory features, and will fire in response to faces, hands, and geometric patterns and objects (Gross et al. 1979; Gross et al. 1972; Sergent et al. 1990), it is possible that not only are auditory and visual memories stored within the inferior temporal lobe, but that it may act as an associational warehouse (so to speak) from which particular auditory-visual images can be activated, retrieved and so that comparisons can be made. Through the neocortex the hippocampus and amygdala can gain access to particular perceptual images as well as store associated information via the aid and guidance of the neural circuits maintained in the entorhinal cortex and through which information is transmitted to both nuclei.
For example, the entorhinal cortex acts as a gateway via which information from the immediately adjacent perirhinal cortex (which sits above and is richly interconnected with the amygdala) and the parahippocampal gyrus (which receives visual, auditory and tactual neocortical information) is analyzed and is then transmitted into the hippocampus (see Horel et al. 2007; Issausti et al. 2007; Squire, 2002). It is also via the entorhinal area that the hippocampus receives amygdaloid projections (Carlsen et al., 1982; Gloor, 1955, 2011; Krettek & Price, 1976; Steward, 1977) and fibers from the orbital frontal and temporal lobes (Van Hoesen, et al., 1972). These neocortical regions are thus highly important in memory.
Squire (2002 p. 202) in fact argues that "the cortical structures adjacent to the hippocampus (entorhinal, perirhinal, and parahippocampal cortex) appear to participate with the hippocampus in a common memory function." He bases this argument on his review of numerous studies (many of which were conducted by Squire and Zola Morgan and colleagues) which indicate that severe memory impairments can result from lesions restricted to these cortical areas.

TEMPORAL LOBE INJURIES & MEMORY LOSS
Although a variety of neurochemical and neuroanatomical regions are involved in the formulation of memory, it has long been known that damage or the neurosurgical removal of the temporal lobes can produce profound disturbances in the learning and recollection of verbal and visual stimuli (Milner, 1958; Kimura, 1963; Nunn et al., 2008; Ploner et al., 2008; Squire, 2007, 2002). For example, left temporal lobectomy, siezures or lesions involving the inferior temporal areas can moderately disrupt immediate and severely impair delayed memory for verbal passages, and the recall of verbal paried-associates, consonant trigrams, word lists and number sequences (Delaney et al. 1980; Meyer, 1959; Meyer & Yates,1955; Milner, 1958, 1968; Milner & Teuber, 1968; Weingartner, 1968). Similarly, severe anterograde and retrograde memory loss for verbal material has been noted when the anterior and posterior temporal regions (respectively) are electrically stimulated (Ojeman et al., 1968, 1971).
In contrast, right temporal lesions or lobectomy significantly impair recognition memory for tactile and recurring visual stimuli such as faces and meaningless designs, as well as memory for object position and orientation, and visual-pictorial stimuli (Corkin, 1965; Delaney et al., 1980; Evans et al. 1995; Kimura, 1963; Milner, 1968; Nunn et al., 2008; Ploner et al., 2008; Taylor, 1969). Electrical stimulation of the right anterior and posterior temporal region also causes respectively, severe anterograde and retrograde memory loss for designs and geometric stimuli, and impairs memory for faces Fried et al., 1982; Ojeman et al., 1968).
With bilateral removal of the inferior temporal region there results a condition which has been variably referred to as "psychic blindness" and the "Kluver-Bucy syndrome". However, as explained in chapter 13, this is due to destruction of the amygdala. If the mesial regions are removed, severe memory disturbances involving visual and auditory stimuli result such that the patient suffers a permanent anterograde amnesia, including facial processing impairments (Young et al. 1995).
Based on lesion, temporal lobectomy and electrical stimulation studies it thus appears that the anterior temporal region is more involved in initial consolidation storage phase of memory, whereas the posterior region is more involved in memory retrieval and recall. In addition, as based on event-related functional magnetic imaging (Brewer et al., 1998; Wagner et al., 2008), it appears that the temporal lobes directly interact with the frontal lobes in memorization and remembering. Indeed, the greater the activation of the frontal and temporal lobes (and associated tissues), the greater is the likelihood that subjects will remember whereas reduced activity is associated with forgetting. Hence, these areas interact to promote memory and retention. In consequence, if the frontal lobe is injured, and even if the temporal lobes are spared, patients may demonstrate significant memory loss --due to an inability to correctly search for and find the memory.

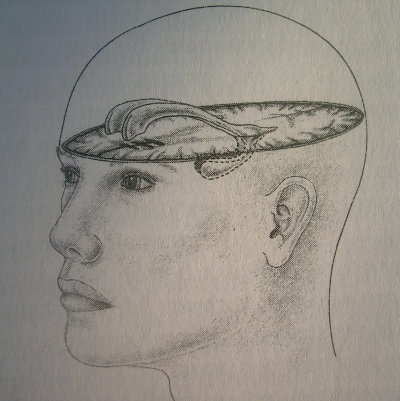
Bilateral destruction of the anterior hippocampus results in striking and profound disturbances involving memory and new learning (i.e. anterograde amnesia). For example, one such individual who underwent bilateral destruction of this nuclei (H.M.), was subsequently found to have almost completely lost the ability to recall anything experienced after surgery. If you introduced yourself to him, left the room, and then returned a few minutes later he would have no recall of having met or spoken to you. Dr. Brenda Milner has worked with H.M. for almost 20 years and yet she is an utter stranger to him.
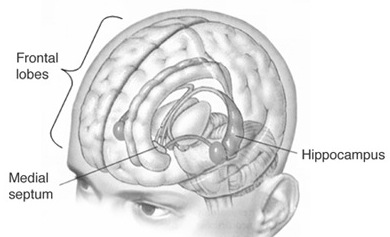
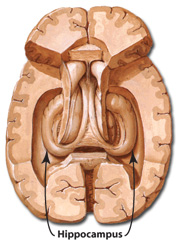
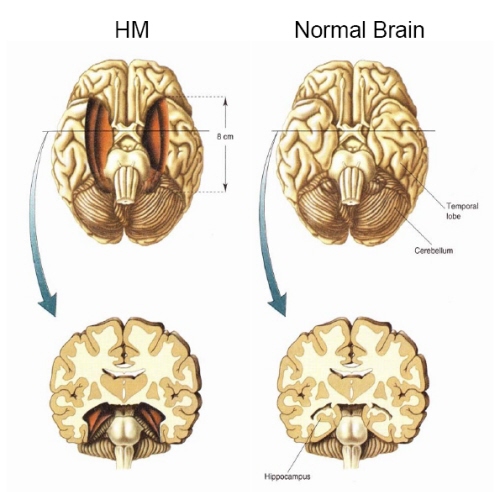
H.M. is in fact so amnesic for everything that has occurred since his surgery (although memory for events prior to his surgery is comparatively exceedingly well preserved), that every time he rediscovers that his favorite uncle died (actually a few years before his surgery) he suffers the same grief as if he had just been informed for the first time.
H.M., although without memory for new (non-motor) information, has adequate intelligence, is painfully aware of his deficit and constantly apologizes for his problem. "Right now, I'm wondering" he once said, "Have I done or said anything amiss?" You see, at this moment everything looks clear to me, but what happened just before? That's what worries me. It's like waking from a dream. I just don't remember...Every day is alone in itself, whatever enjoyment I've had, and whatever sorrow I've had...I just don't remember" (Blakemore, 1977, p.96).
Presumably the hippocampus acts to protect memory and the encoding of new information during the storage and consolidation phase via the gating of afferent streams of information and the filtering/exclusion (or dampening) of irrelevant and interfering stimuli. When the hippocampus is damaged there results input overload, the neuroaxis is overwhelmed by neural noise, and the consolidation phase of memory is disrupted such that relevant information is not properly stored or even attended to. Consequently, the ability to form associations (e.g. between stimulus and response) or to alter preexisting schemas (such as occurs during learning) is attenuated (Douglas, 1967).
HALLUCINATIONS
The functional integrity of the temporal lobes, the inferior regions in particular, are highly important in regard to the memorization and recollection of various auditory, visual, olfactory, and emotional experiences. When destroyed, disconnected from sources of input, or compromised in some fashion, the ability to store information and to draw visual-verbal mnemonic imagery from memory is severely attenuated.
Conversely, when the temporal lobes and/or the limbic nuclei buried within its depths (i.e. the amygdala and hippocampus) are artificially or abnormally activated it sometimes occurs that visual-auditory imagery as well as a variety of emotional reactions are evoked involuntarily. These may take the form of complex hallucinations, dream-like states, confusional episodes, or may involve the abnormal attribution of emotional significance to otherwise neutral thoughts and external experiences.
HALLUCINATIONS & THE INTERPRETATION OF NEURAL "NOISE".
Hallucinations may occur secondary to tumors or seizures involving the occipital, parietal, frontal, and temporal lobe (Gloor, 2011; Halgren, 2002; Penfield & Perot, 1963), or arise secondary to toxic exposure, high fevers, general infections, exhaustion, starvation, extreme thirst, partial or complete hearing loss including otosclerosis, and with partial or complete blindness such as due to glacoma (Bartlet, 1951; Flournoy 1923; Lindal et al. 2014; Pesme, 1939; Rhein, 1913; Ross et al., 1975; Rozanski & Rosen, 1952; Semrad, 1938; Tarachow, 1941). Interestingly, when secondary to peripheral hearing loss, frequently individuals report hearing certain songs and melodies from their childhood --melodies which they had usually long forgotten. In addition, individuals suffering from cortical blindness, i.e. Anton's syndrome (Redlich & Dorsey, 1945) and deafness (Brown, 1972), as well as those recovering from Wernicke's aphasia, frequently experience hallucinations.
In general, hallucinations secondary to loss of visual or auditory input appears to be secondary to the interpretation of neural noise. That is, with loss of input various brain regions begin to extract or assign meaningful significance to random neural events, or to whatever input may be received. Thus we find that subjects will hallucinate when placed in sensory reduced environments or even when movement is restricted (Lilly, 1956, 1972; Lindsley, 1961; Shurley, 1962; Zuckerman & Cohen, 1964).
Conversely, hallucinations can occur due to increased levels of neural noise as well. For example, if an area of the neocortex is abnormally activated that area in turn may act to interpret its own neural activity, and/or as a function of the activation of "feature detectors." For example, those neurons that subserve facial recognition, and word recognition, and object recognition, may become simultaneously activated--as well as all associated memories--and in consequence, the brain attempts to interpret what it experiences.
However, the degree of interpretative activity depends on the type of processing performed in the region involved. In this regard we find that hallucinations become increasingly complex as the disturbance expands from primary to association areas and as involvement moves from the occipital to anterior temporal regions (Critchley, 1939; Penfield & Perot, 1963; Tarachow, 1941) --which is one of the major interpretive regions of the neocortex (Gibbs, 1951; Gloor 1990, 2002; Halgren 2002; Penfield & Perot, 1963). That is, in the primary regions, neural noise is given a simple interpretation (simple hallucinations), whereas in the association and multi-associational areas, the individual begins to hallucination secondary to "feature detector" activation, such that they may see faces, chairs, trees, hear voices, music, and so on, all of which is experienced as a mosaic of something real.
For example, tumors or electrical stimulation of the occipital lobe produce simple hallucinations such as colors, stars, spots, balls of fire, flashes of light, whereas with superior temporal involvement the patient may experience crude noises, such as buzzing, roaring sounds, bells, and an occasional voice or sounds of music. However, these same neurons become activated when presented with colors, spots, flashes of light, bells, and so on.
However, with anterior and inferior temporal abnormalities, the hallucinations become increasing complex consisting of both auditory and visual features, including faces, people, objects, animals, etc. (Critchley, 1939; Penfield & Perot, 1963; Tarachow, 1941). This is because there is a ventral stream of visual experience which becomes increasingly complex as information is transmitted from the primary to secondary to association, to multi-modal areas in the temporal lobes where neurons fire in response to complex objects, including faces. The anterior-inferior temporal regions, therefore, give rise to the most complex forms of imagery because cells in this area are specialized for the perception and recognition of specific forms. Moreover, structures such as the amygdala and hippocampus become activated and in consequence, memories and emotions may also be evoked, such that the experience may also become personally meaningful and include real individuals and real events that are produced from memory (Gloor, 2011; Penfield & Perfot, 1963).
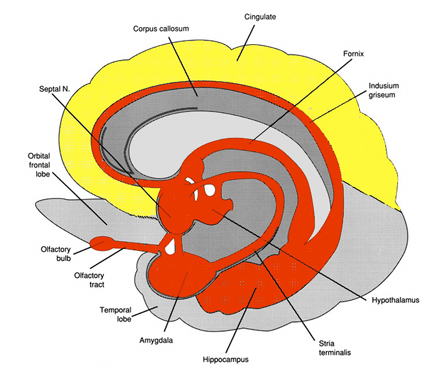
It has frequently been reported that as compared to other cortical areas, the most complex and most forms of hallucination occur secondary to temporal lobe involvement (Critchley, 1939; Malh et al., 1964; Horowitz et al., 1968; Penfield & Perot, 1963; Tarachow, 1941) and that the hippocampus and amygdala (in conjunction with the temporal lobe) appear to be the responsible agents (Gloor 1990, 2002, 2011; Gloor et al., 1982; Horowitz et al., 1968; Halgren et al., 1978). For example, Bancaud et al. (2014), Halgren et al., (1978), and Horowitz and colleagues (1968) note that hippocampal stimulation was predominatly associated with either fully formed and/or memory-like hallucinations including feelings of familiarity, and secondarily dream-like hallucinations. However, stimulation limited to the neocortex generally failed to produce complex hallucinations unless the amygdala became activated (Gloor et al., 1982). In this regard, it appears that limbic activation, and activation of the amygdala in particular (Gloor, 2011) is necessary in order to bring to a conscious level percepts which are being processed in the temporal lobes.
LSD. This does not mean, however, that neocortical involvement is not necessary for frequently it is the interpretive interaction of the temporal lobe which gives rise to certain types of hallucinations; i.e. bringing them to a conscious level. That is, although the amygdala (perhaps acting on the hippocampus) may be responsible for the hallucination, the hallucination requires an interactions between the amygdala, hippocampus, and the neocortex, for it is only when the "hallucination" envelops the neocortex of the temporal lobe that the individual becomes "conscious of them."
For example, it is well known that the ingestion of LSD will trigger the formation of vivid and complex auditory and visual hallucinations. Following LSD administration electrophysiological abnormalities are noted in the amygdala and hippocampus (Chapman et al., 1963). However, if the temporal lobes are surgically removed there is a significant decrease (with unilateral removal, Serafetinides, 1965) or complete abolishon (with bilateral temporal lobe removal) of LSD induced hallucinatory activity (Baldwin et al., 1955)--even when the amygdala and hippocampus are spared. That is, if the overlying neocortex is destroyed the individual will fail to hallucination--or at least to become conscious of the hallucination. Of course, if the overlying temporal lobe is destroyed the amygdala and hippocampus also become disconnected, and cannot help but be injured.
Nevertheless, studies with LSD and temporal lobe neocortical resection suggest that the neocortex is associated with consciousness and the limbic system with the unconscious, and if the pathways between them are destroyed, hallucinations cease to be experienced consciously.
Interestingly, the hallucinatory effect of LSD appears to be greatest in the right temporal lobe (Serafetinides, 1965). That is, destruction of the right temporal lobe abolishes LSD-induced hallucinations, where with left temporal destruction, the individual continues to hallucination. Likewise, Penfield and Perot (1963) report that the most vivid hallucination tend to be triggered from the right not the left temporal lobe.

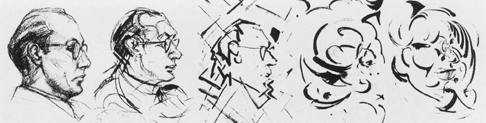
DREAMING
Vivid, visual-auditory and sometimes intensely emotional hypnogic dream imagery is clearly associated with REM sleep (Foulkes, 1962; Goodenough, Shapiro, Holden, & Steinschriber,1959; Monroe, Rechtschaffen, Foulkes, & Jensen, 1965). As discussed in detail in Chapter 10, electrophysiological studies or measures of cerebral blood flow have indicated that the right hemisphere becomes highly active during REM, whereas conversely the left brain becomes more active during N-REM (Goldstein et al., 1972; Hodoba, 1986; Meyer et al., 2007).
It has also been reported that abnormal and seizure-like activity in the right temporal and temporal-occipital area acts to increase dreaming and REM sleep for an atypically long time period. Similarly, REM sleep increases activity in this same region much more than in the left hemisphere (Hodoba, 1986) indicating that there is a specific complementary relationship between REM sleep, dreaming, and right temporal-occipital electrophysiological activity.
In this regard, although not conclusive, there seems to be a convergence of evidence which suggests that the right temporal lobe may be more involved than the left in the production of visual-auditory hallucinations and dream-like mental states be they produced secondary to LSD, electrical stimulation, abnormal seizure activity, or occurring naturally during sleep.
As pertaining to right vs. left cerebral involvement in the production and recollection of hallucinations and dreams it is interesting to note that Horowitz et al., (1968), reported that electrically induced hallucinated events were usually forgotten by patients within 10 to 15 minutes after the experience, and when questioned the next day memory was not improved. Similar forgetting patterns are characteristic of memory for normal sleep induced dreams as well (Joseph, 1988a). That is, it becomes progressively more difficult to recall one's dreams as one spends time in or awakens during NonREM (Wolpert & Trosman, 1958) -which is associated with high left temporal lobe and low right lobe activitity.
TEMPORAL LOBE (PARTIAL COMPLEX) SEIZURES & EPILEPSY
Temporal lobe epilepsy is often associated with atrophy and sclerosis of the hippocampus in about 50% of such patients (Mathern, 2008; Mitchell et al., 2008), with yet others demonstrating sclerosis in the amygdala (Wolf & Blumcke 2008). In fact, according to Gloor (2011, p. 692), "studies on temporal lobe epilepsy provide evidence that the amygdala is involved inmany of the common symptoms and signs that occur in the course of temporal lobe seizures." Gloor (2011, p. 693), also argues that "hippocampal sclerosis is most likely a consequence of prolonged seizures or status epilepticus, particularly when occurring in early childhood and can therefore be regarded as a lesion induced by seizures rather than a causative agent." Gloor (2011) further suggests that the seizure is either produced by the amygdala, which then envelops the hippocampus, and/or that both must give rise to the seizure simultaneously. In support of this position are the findings of Feindel and Rasmussen (2011) who report 65 of 100 neurosurgery epilepsy patients in whom the amygdala and the hippocampus was removed showed a favorable outcome, whereas an equal number showed a favorable outcome if just the amygdala was removed and the hippocampus was spared.
Because the hippocampus and amygdala have the lowest seizure thresholds of all other brain structures, when injured they are thus highly likely to develop seizure activity and thus give rise to temporal lobe epilepsy.
There are a number of factors which can independently give rise to temporal lobe epilepsy (Kotagal & Luders, 2008; Mathern, 2008; Mitchell et al., 2008; Trimble, 2011; Wolf & Blumcke 2008). For example, incisural hernation at the time of brith is a major cause of amygdala injury and hippocampal sclerosis. Head injuries also cause injuries to the temporal region due to contusion from the bony structures in which it is encased. That is, if the head is struck, the inferior and anterior temporal regions may strike against the skull and may even be sheared due to rotational forces. Also middle ear infections are associated with disturbances involving the temporal lobe which result in TLE (Kotagal & Luders, 2008).
In addition, as detailed in chapter 30, severe and repeated early emotional trauma can in fact injure the immature hippocampus and temporal lobe, giving rise to a propensity to develop kindling as well as abnormal neural networks. This would make these individuals are more likely to develop psychotic and severe emotional and dissociative disorders.
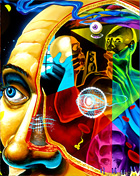
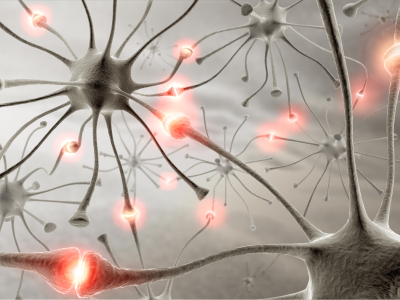
Usually, during the course of a temporal lobe seizure, there are no abrupt and drastic alterations in motor activity such as tonic-clonic spasms (Kotagal & Luders, 2008; Trimble, 2011, 1996). However, some patients may simply cease to respond and stare blankly straight ahead, make licking or smacking movements of the lips, and/or fiddle with their clothing, as if picking up pieces of lint. Although conscious-awareness is lost, these patients are not unconscious. Rather, their mental state is one of absence. In fact, they may appear awake and conscious, although unable to speak or respond to questions, and behaviorally their actions seem semi-purposeful. Nevertheless, sometimes it is extremely obvious that they are experiencing a seizure, whereas in some cases an inexperienced observor may only have the impression that the person is acting somewhat odd.
Nevertheless, unless the patient has several different seizure foci, the same characteristic behavioral manifestations are elicited every time a patient has a seizure. The patient does not simply stare on one occasion and on the next begin rolling his eyes and cry out.
AURAS & AUTOMATISMS
Some patients also note an aura immediately prior to seizure onset (Kotagal & Luders, 2008; Trimble, 2011, 1996). This may involve feelings of fear or anxiety, alterations in gastric motility, or unpleasant tastes or in particular, odors (e.g. burning rubber or feces), i.e. an olfactory aura (Gloor, 2011). Some patients may lick and repeatedly smack their lips.
Presumably, the experience of olfactory hallucinations is due to abnormal activation of the rhinencephalon (the "nose brain") and thus limbic nuclei such as the amygdala (Gloor, 2011). Possibly the licking and smacking movements are also due to activation of the amygdala and other limbic structures associated with food consumption. Changes in gastrict motility may be secondary to insular activation and/or limbic participation.
Automatisms associated with TLE occur in up to 75-95% of patients which is twice what occurs with lesions outside the temporal lobe (Kotagal & Luders, 2008; Trimble, 2011, 1996). These includes staring, searching, groping, lip smacking, spitting, groping, staring, salivation. Also laughing, cryng, hissing, gritting or gnashing of teeth, clenching the fist, confused talking, screaming, shouting, standing, running, walking, kissing, and so on.
Common visceral reactions associated with TLE auras include feelings of a racing or flutering heart, incuding pounding or throbbing (Kotagal & Luders, 2008; Trimble, 2011, 1996) also there may be feelings of smothering or chocking. Body sensation include numbness, tenseness, pressure and heaviness. Ther may also be visual sensations such as macro or micro or of things be very hear or far (Gloor, 2011).
Feelings of strangeness or familiarity are also common (Gloor, 2011; Kotagal & Luders, 2008; Trimble, 2011, 1996). deja vue occurs in up to 20% of such patients, usually with right cerebral seizures within the temporal lobe. Some claim to have feelings like a desire to be alone, or of wanting something but not knowing what.
Olfactory hallucinations are not uncommon and are usually quite disagreeable and include smells like burning meat, fish, lime, acid fumes, burning shit. There may also be gustatory sesatniosn which are also usually disagreeable coupled with very bad and bitter or metalic and sour tastes.
Fear is one of the most common feelings, which include feelings of panic, terror, or of something horrible about to happen (Daly, 1958; Gibbs, 1958; Penfield & Jasper, 1954; Williams, 1956). Some patients have experienced fear coupled with sexual sensations, including fear associated with a feeling of sexual climax, or feelings of being sexually penetrated following by orgasm then a loss of consciousness. Although one patient stated it was like "a red hot pocker being inserted into her vagina."
Hence, there are a vast range of aura and experimental phenomenon associated with temporal lobe epilepsy, many of which reflect the possible activation of those structures innervated by the amygdala. Not uncommonly these experiential phenomenon begin just before the seizure. In summary, these include fear, coupled with the remembrance of a fearful or traumatic memories, rising epigastric sensations, chest sensations, nausea, heart palpations, feelings of cold or warmth, shivering, pallor or flushing of the face, respiratory changes inlcuding apnea, salavation, belching, farting, sweating, and vaginal secretions in women which may be accompanied by sexual feelings or behaviors (Daly, 1958; Gloor, 2011; Penfield & Jasper, 1954; Remillard et al., 1983). Moreover, many of these same exact feelings and behaviors can be triggered by direct stimulation of the amygdala (Chapman, 1960; Chapman et al., 1954; Gloor, 2011; Halgren, 2002; Kaada, 1951, 1972), including deja vu--the feeling of familiarity/reminiscence.
In addition, patients may act out on these emotions and auras. There have been reported instances of patient's suddenly lashing out and even attempting to attack those close by, while in the midst of a seizure (Saint-Hilaire et al., 1980), and/or attacking, kicking, and destroying furniture and other objects (Ashford et al., 1980). Patient's have also behaved violently with direct stimulation of the amygdala (Mark et al., 1972), as have a variety of animal subjects (see chapter 13).
Others have responded with behaviors indicative of extreme fear, or conversely, extreme pleasure, including laughter and mirth. Moreover, some patients have acted out sexually during a seizure, such as by displaying their penis, or lying on the floor with legs spread and thrusting, even moaning with pleasure and experiencing libidinous feelings in the vagina and thighs, coupled with an orgasm (Currier, Little, Suess and Andy, 1971; Gloor, 2011; Remillard et al., 1983).
to develop psychotic and severe emotional and dissociative disorders.
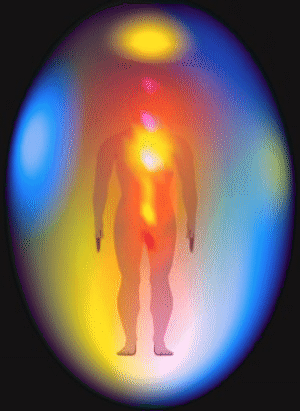
SEXUAL SEIZURES
The primate amygdala is sexually differentiated (Nishizuka & Arai, 2011) and in conjunction with the overlying temporal lobe, is capable of detecting sexually significant stimuli, and can determine and detect gender differences such as male and female faces and the emotions they convey (Hasselmo, Rolls, & Baylis, 2008, Heit et al., 1988; Rolls, 2004). Because the amygdala is involved in sexuality and is sexually differentiated, activation of the amygdala can produce penile erection (Kling and Brothers, 2002; MacLean, 1990; Robinson and Mishkin, 1968; Stoffels et al., 1980) sexual feelings (Bancaud et al., 1970; Remillard et al., 1983), sensations of extreme pleasure (Olds and Forbes, 2011), memories of sexual intercourse (Gloor, 1986), as well as ovulation, uterine contractions, lactogenetic responses, and orgasm (Backman and Rossel, 2004; Currier et al., 1971; Freemon and Nevis,1969; Warneke, 1976; Remillard et al., 1983; Shealy and Peel, 1957).
By contrast, injuries to and/or seizure activity within the amygdala/temporal lobe may result in bizarre sexual changes, such as continuous masturbation and indiscriminate, often hypersexual hetero- and homosexual behaviors including attempts at sex with inanimate objects (Kling and Brothers, 2002; Kluver and Bucy, 1939; Pribram and Bagshaw 1953; Terzian and Ore, 1955). Hypersexuality following amygdala injury has been documented among numerous species, including cats and dogs (Blumer 1970; Kling and Brothers, 2002).
Humans with an abnormally activated or severely injured amygdala/temporal lobe may expose and manipulate their genitals (Leutmezer et al., 2008), masturbate in public, and attempt to have sex with family members or individuals of the same sex (Blumer, 1970; Kolarsky, Freund, Macheck, and Polak, 1967; Terzian and Ore, 1955). Moreover, abnormal activity involving the amygdala and overlying temporal lobe has been associated with the the development of hyposexuality (Taylor, 1971; Heirons and Saunders, 1966; Toon, Edem, Nanjee, and Wheeler, 2008), hypersexuality (Blumer, 1970) as well as homosexuality, transvestism, and thus confusion over sexual orientation (Davies and Morgenstern, 1960; Kolarsky et al., 1967). In fact, abnormal- or seizure activity within the amygdala or overlying temporal lobe may induce an individual to engage in "sexual intercourse" even in the absence of a partner. For example, Currier and colleagues (1971, p. 260) described a female temporal lobe seizure patient who was "sitting at the kitchen table with her daughter making out a shopping list" when she suffered a seizure. "She appeared dazed, slumped to the floor on her back, lifted her skirt, spread her knees and elevated her pelvis rhythmically. She made appropriate vocalizations for sexual intercourse such as: It feels so good...further, further."
RUNNING, LAUGHING & CRYING SEIZURES
In some instances patients with temporal lobe seizures (triggered perhaps by tumor) may display extremely odd and bizzare behaviors during the course of the seizure (Chen & Forster, 1973; Trimble 2011) For example, a number of authors have presented case reports of individuals who developed laughing (gelastic epilepsy), crying (dacrystic epilepsy), and/or running seizures (cursive epilepsy) -running apparently being triggered via amygdala-striatal activation (chapter 13, 16).
One patient's seizure consisted of suddenly bursting into laughter, rubbing his upper abdomen and running wildly about the room with an expression of fear on his face (Sethi & Rao, 1976). Another individual displayed paroxysmal attacks of weeping, sobbing, and mournful moaning (Offen et al., 1976). These behaviors were involuntary, however, and part of the seizure. Once consciousness returned, the patients would have no recollection of their actions.
EMOTION
The most common emotional reactions and sensations which occur secondary to or during the course of a temporal lobe seizure include feelings of fear, anxiety, depression, depersonalization, pleasure, unpleasure, and familiarity (Bear, 1979; Cendes, et al. 2014; Herman & Chhabria, 1980; Gloor, 2011; Gloor et al., 1982; Perez & Trimble, 1980; Trimble 2011; Slater & Beard 1963; Strauss et al., 1982; Weil, 1956; Williams, 1956), fear being the most frequently experienced. Many of these same feelings are also triggered by electrical stimulation of the temporal lobes, amygdala and hippocampus (Gloor, 1972, 2011; Gloor et al., 1982; Heath, 1964; Mullan & Penfield, 1959).
Depression (lasting from hours to weeks) may occur as an immediate sequela to the seizure, many patients also experiencing confusion. A depressive aura may also precede and thus hearald the coming of a seizure by hours or even days (Trimble 2011, 1996; Williams, 1956). This may be due in part to the role of the temporal lobe (and amygdala) is storing emotional and personal memories, including those with are negative, depressing and traumatic (Abrams & Taylor, 1979; Cimino, et al. 2011 Cohen, Penick & Tarter, 1974; Deglin & Nikolaenko, 1975; Shagass et al.,1979; Wexler, 1973) such that when activated, depressive moods and memories may be provoked. For example, Abrams and Taylor (1979) and Shagass et al. (1979) have found that depressed patients demonstrated more right vs left temporal lobe electrophysiological activity and EEG abnormalities. Moreover, others have argued that ECT administered to the right vs left temporal lobe is more likely to alleviate depressive symptoms (Cohen, et al, 1974; Deglin & Nikolaenko, 1975).
Rather than increased emotionality, some patients complain of emotional blocking, and feelings of emptiness: "feelings don't reach me anymore" (Weil, 1956). Presumably this is a consequence of limbic disconnection. That is, the seizure foci (or lesion) acts to deconnect the limbic areas from the temporal (or orbital frontal) lobes. In consequence, percepts and thoughts no longer come to be assigned emotional or motivational significance.
TEMPORAL LOBE EPILEPSY & RELIGIOUS EXPERIENCE
It has been reported by a number of neuroscientists that some patients who experience temporal lobe epilepsy, seizures, or related abnormalities sometimes experience very sexual as well as bizarre, unusual and fearful mental phenomenon including dissociative states, feelings of depersonalization, and hallucinogenic and dream-like recollections involving threatening men, naked women, sexual intercourse, religion, the experience of god, as well as demons and ghosts and pigs walking upright dressed as people (Bear 1979; Daly 1958; Gloor 1986, 2002; Halgren 2002; Horowitz et al. 1968; Penfield & Perot 1963; Slater & Beard 1963; Taylor 1972 1975; Trimble 2011; Weingarten, et al. 1977; Williams 1956). Moreover, some individuals report communing with spirits or receiving profound knowledge from the Hereafter, following temporal lobe activation (Daly 1958; MacLean 1990; Penfield & Perot 1963; Williams 1956).
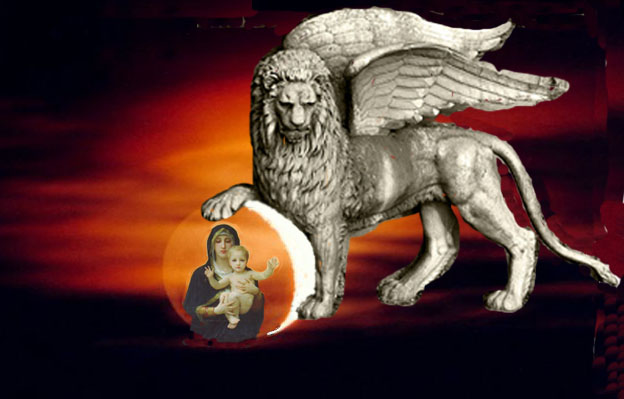
One young many had repeated conversations with God. One day God informed this young man that he was Jesus Christ, could perform miracles and could fly. Believing that he was in fact The Christ, since god had told him so, he climbed upon a roof and leaped into the air, with disasterious results (Sommer 1880).
Intense activation of the temporal lobe, hippocampus, and amygdala has been repeatedly reported to give rise to a host of sexual, religious and spiritual experiences; and chronic hyperstimulation can induce an individual to become hyper-religious or visualize and experience ghosts, demons, angels, and even God, as well as claim demonic and angelic possession or the sensation of having left their body (Bear 1979; Daly 1958; Gloor 1986, 2002; Horowitz et al. 1968; MacLean 1990; Mesulam 2011; Penfield & Perot 1963; Schenk, & Bear 2011; Slater & Beard 1963; Subirana & Oller-Daurelia, 1953; Trimble 2011; Weingarten, et al. 1977; Williams 1956). Indeed, as detailed in chapter 9, the temporal lobe, amygdala and hippocampus enables humans to have religious, spiritual and mystical experiences (Bear 1979; Daly 1958; d'Aquili & Newberg 1993; Mesulam 2011; Trimble 2011).
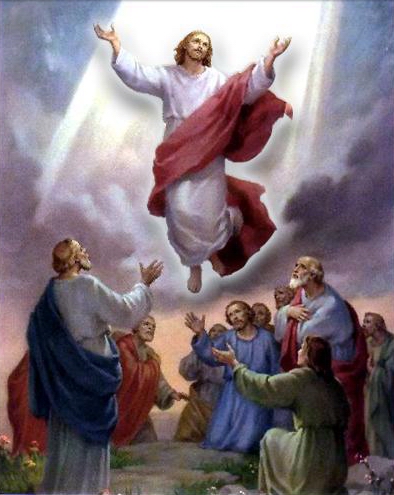
In one case, a 37 year-old manager of a car dealership had been struck by a car while walking across a street, and was slammed against the pavement, striking his head. According to this fellow (GM), once he gained "consciousness" he realized he was standing next to his body, which was still lying in the street. He could see that his head was bloody, and he could see that he was unconscious and he could see other people rushing over to where he lay. However, this dissociative hallucination only lasted a few seconds, and then he was back in his body, regaining consciousness. GM states that his experience was a "revelation" and although he had never been religious, that he became very religious, and began reading the Bible, volunteering at a local church, and writing a religious column for a small local newspaper. He also began having repeated episodes where he would float beside his body. As his wife complained that he was repeatedly "blanking out" and as he was also having memory problems, he sought medical help, was referred for neuropsychological testing, which revealed significant disturbances of memory, and evidence of temporal lobe epilepsy. Subsequent EEG demonstrated bilateral spiking and theta activity over the temporal lobes.
OUT OF BODY, HEAVENLY & "OTHERWORLDLY EXPERIENCES"
Penfield and Perot (1963) describe several patients who during a seizure claimed they could see themselves in different situations. One woman stated that "it was though I were two persons, one watching, and the other having this happen to me," and that it was she who was doing the watching as if she was completely separated from her body. According to Penfield, "It was as though the patient were attending a play and was both actor and audience.
Other patients claim to have quite pleasant auras and describe feelings such as elation, security, eternal harmony, immense joy, paradisiacal happiness, euphoria, completeness. Between .5 and 20% of such patients claim such feelings (Williams, 1956; Daly, 1958). One patient of Williams (1956) claimed that his attacks began with a "sudden feeling of extreme well being involving all my senses. I see a curtain of beautiful colors before my eyes and experience a pleasant but indescribable taste in my mouth. Objects feeling pleasurably warm. the room assumes vast proportions, and I feel as if in another world."
A patient described by Daly said his seizure felt like "a sunny day when your friends are all around you." He then felt dissociated from his body, as if he were looking down upon himself and watching his actions.
Williams (1956) describes a patient who claimed that during an aura she experienced a feeling that she was being lifted up out of her body, coupled with a very pleasant sensation of elation and the sensation that she was "just about to find out knowlegde no one else shares, something to do with the line between life and death."
Subirana and Oller-Daurelia (1953) described two patients who experienced ecstatic feelings of either "extraordinary beatitude" or of paradise as if they had gone to heaven and noted that his fantastic feelings lasted for hours.
Other patients have noted that feelings and things suddenly become "crystal clear" or that they have a feeling of clairvoyance, or of having the truth revealed to them, or of having achieved a sense of greater awareness and of a new awareness such that sounds, smells and visual objects seemed to have a greater meaning and sensibility.
PERSONALITY & PSYCHIATRIC DISTURBANCES
Personality and psychiatric disorders have been frequently reported as a common associated complication among a small percentage of individuals (between 4.5 to 7%) with seizures-disorders localized to the temporal lobe (Harrison, 2008; Trimble 2011, 1996). Such patients may appear depressed, paranoid, hysterical, or suffering from schizophrenic-like symptomology (However, see Gold et al. 2014).
Some authors have argued that, like hallucinations, the nearer the seizure foci to the anterior temporal lobe, the greater the probability of significant psychiatric abnormality (Gibbs, 1951; Gloor 2002; Trimble 2011, 1996). Presumably this is a function of hyper-activation of underlying limbic structures and thus abnormal attribution of emotional significance to different afferent streams of perceptual experience (Bear, 1979).
SCHIZOPHRENIA & THE TEMPORAL LOBE
It has been repeatedly reported that a significant minority of individuals with temporal lobe epilepsy suffer from prolonged and/or repeated instances of major psychopathology (Bruton et al. 2014; Buchsbaum, 1990; Falconer, 1973; Flor-Henry, 1969, 1983; Gibbs, 1951; Jensen & Larsen, 1979; Perez & Trimble 1980; Sherwin, 1977, 2011; Slater & beard, 1963; Taylor, 1972; Trimble 2011, 1996). Conversely, those with affective disturbances, e.g. mania, have been found to suffer from seizures involving the right temporal lobe (Bear et al., 1982; Falconer & Taylor, 1970; Flor-Henry, 1969; Trimble 2011, or right frontal lobe (chapter 19), although depression may be more likely with left vs right hemisphere injuries (see chapter 11).
Estimates of psychosis and affective disorders among individuals suffering from temporal lobe epilepsy, be it a single episode or chronic psychiatric condition have varied from 2-81%. Probably a more realistic figure is 3% to 7% (see Trimble, 2011, 2002, 1996).
There are several distinct neurological explanations for the association between temporal lobe damage and psychotic and affective disorders. As discussed in detail in previous chapter 10, the right hemisphere is dominant for most aspects of emotional functioning. Hence, when damaged or abnormally activated, such as from a seizure or chronic subclinical seizure activity, emotional functioning becomes altered and disturbed. Moreover, since the amygdala and hippocampus are often secondarily aroused (or hyperaroused), the possibility of abnormal emotionality is enhanced even further.
In this regard, Gloor et al., (1982) in a depth electrode stimulation study of patients suffering from epilepsy presented evidence indicating that all experiential and emotional alterations were encountered following right amygdala and hippocampal activation, with the majority of phenomenon elicited from the amygdala. As discussed in chapter 13, the limbic system seem to be lateralized and the right amygdala and hippocampus appear to be more involved in emotional functioning . Hence, abnormal activation of these nuclei (such as during the course of a temporal lobe seizure) are more likely to give rise to abnormal emotional reactions, with the exception of depression which is common with left frontal and left temporal lobe injuries.
In addition, some individuals with temporal lobe seizures may complain of emotional blunting. Hence, rather than a limbic hyperconnection, these individuals suffer from a disconnection; similar to what occurs with a left frontal injury.
It has also been repeatedly demonstrated in histological studies that the temporal lobe of schizophrenics are characterized by an excessive focal temporal lobe neuronal damage including gliosis (Akbarian et al. 1993; Bruder, et al., 2008; Bruton 1988; Bruton et al. 1990; Harrison, 2008; Kwon et al., 2008; Stevens 1982; Taylor 1972). Hence, these patients may experience auditory hallucinations and are more likely to demonstrate formal thought disorders and aphasic thinking including the production of neologisms and syntactical speech errors (Kwon et al., 2008), as well as emotional (or thought) blocking or blunting due to limbic system-temporal lobe disconnection (Perez & Trimble, 1980; Slater & Beard, 1963). As often occurs with receptive aphasia and left temporal lobe dysfunction, individuals suffering from schizphrenia may be unaware of their illness (Amador et al. 2014; Lysaker, et al., 2008; Wilson et al. 1986).
Hence, certain subgroups of schizophrenics display significant abnormalities involving speech processing, such that the semantic, temporal-sequential, and lexical aspects of speech organization and comprehension are disturbed and deviantly constructed (Chaika, 1982; Clark et al. 2014; Flor-Henry, 1983; Hoffman, 1986; Hoffman et al. 1986; Rutter, 1979). Indeed, significant similarities between schizophrenic discourse and aphasic abnormalties have been reported (Faber, Abrams, Taylor, Kasprisin et al. 1983). It is often difficult to determine what a schizophrenic individual may be talking about, particularly in that some of these individuals complain that what they say often differs from what they intended to say (Chapman, 1966). Temporal-sequentual (i.e. syntactical) abnormalities have also been noted in their ability to reason. The classic example being: "I am a virgin; the Virgin Mary was a virgin; therefore I am the Virgin Mary."
Hence, there is considerable evidence indicating that a significant relationship exists between abnormal left hemisphere and left temporal lobe functioning and schizophrenic language, thought and behavior. As detailed in chapter 19, the left frontal region and medial frontal cortices are also implicated in the genesis of schiophrenic-like abnormalities including catatonia (chapter 19).
However, in contrast to those with frontal lobe schizophrenia who are more likely to present with "negative" symptoms and emotional blunting, temporal lobe schziophrenics are likely to present with "positive" symptoms, including retention of the capacity to experience and express "warmth" (Crow, 1980; Crowe & Kuttner 2011). Emotional expression, however, tends to be abnormal, inappropriate or incongruent, and/or exaggerated (Bear & Fedio, 1977; Crow, 1980; Crowe & Kuttner 2011).
It has also been repeatedly reported that irritative lesions more greatly involves the lateral convexity of the left temporal lobe are he most likely to result in schizophrenic-like disturbances (DeLisi et al. 2011; Dauphinais et al. 1990; Flor-Henry 1983; Harrison, 2008; Perez et al. 2015; Rossi et al. 1990, 2011; Sherwin 2011). Given the role of the temporal lobe in language (and the fact that the superior temporal lobe is derived from amygdala and hippocampal tissue) it is also not uncommon for these individuals to demonstrate aphasic abnormalities in their thought and speech (Chaika, 1982; Clark et al. 2014; Flor-Henry, 1983; Hoffman, 1986; Hoffman et al. 1986; Perez & Trimble, 1980; Rutter, 1979; Slater & Beard, 1963).
However, the type and form of temporal lobe schizophrenia may differ dependent on which regions have been compromised. In some instances the amygdala and hippocampus are involved, (Bogerts et al. 2015; Brown et al. 1986; Falkai & Bogerts 1986) in which case memory and emotional functioning may be most severely effected, and patients may experience visual hallucinations. Temporal lobe schizophrenics with limbic involvement may behave violently and aggressively (Crow & Kuttner 2011; Lewis et al. 1982; Taylor 1969). They may also experience extreme feelings of religiousness (Bear, 1979, Trimble, 2011, 2002), fear, paranoia, and depression (Crow & Kuttner 2011; Perez & Trimble, 1980; Trimble, 2011, 2002), as well as hallucinations.
APHASIA & PSYCHOSIS
Since the left temporal lobe is intimately involved in all aspects of language comprehension as well as the organization of linguistic expression, altered neocortical activity involving this region is likely to result in significant disruptions in language functioning and the formulation of linguistic thought. As described in chapter 11, individuals with left temporal lobe damage and Wernicke's aphasia are likely to be diagnosed as suffering from a formal thought disorder due to their fluent aphasic language disturbances their failure to comprehend.
Conversely, several authors have noted significant similarities between schizophrenic discourse and aphasia (Faber et al. 1983; Hillbom. 1951), such that the semantic, temporal-sequential, and lexical aspects of speech organization and comprehension are disturbed and deviantly constructed (Chaika, 1982; Flor-Henry, 1983; Hoffman, 1986; Hoffman, Stopek & Andreasen, 1986; Rutter, 1979). Similar findings have been reported for individuals developing post-traumatic schizophrenic-like symptoms following head injury and missile wounds (Hillbom, 1951). Moreover, just as some aphasic individuals may be unaware of their disorder, similar findings have been noted with schizophrenics and those with schizoaffective disorders (Amador et al. 2014).
TEMPORAL LOBE SEIZURES, PSYCHOSIS & APHASIA
Patients with left temporal seizures commonly become globally aphasic during the course of their seizure. When spoken to or if their name is repeatedly called they may make only fleeting eye contact, grunt or utter partial words such as "huh?" Some individuals remain aphasic for seconds to minutes after seizure termination as well.
Language impairments, verbal memory disorders and associated linguistic deficiencies are usually apparent even between seizures if adequately assessed. Moreover, individuals with left sided foci tend to have lower (WAIS-R) Verbal IQ's as compared to their Performance IQ's and as compared to those with right sided foci. This is not surprising as language is represented in the left hemisphere. However, in some cases, excessive activity within the left (or right) temporal (or frontal) lobe can actually result in what appears to be enhanced functional activity.
It is possible that abnormal activity in the left temporal lobe, as well as the presence of an active lesion, can result in significant alterations in not only language, but the organization of linguistic thought such that the patient appears psychotic. In this regard, abnormal thought formation may become a characteristic pattern. In fact, even the neuroanatomical representation of language can be drastically altered due to left cerebral damage (Joseph, 1986a; Novelly & Joseph, 1983). Moreover, if the left temporal region is periodically disconnected from centers mediating emotion, patients may demonstrate what appears to be emotional blunting as well as a formal thought disorder.
BETWEEN SEIZURE PSYCHOSES
The range of disturbances among those with temporal lobe epilepsy includes a strikingly high rate of sexual abberation as well as hyposexuality, aggressiveness, paranoia, depression, deepening of emotion, intensification of religious concerns, disorders of thought, depersonalization, hypergraphia, complex visual and auditory halluciantions and schizophrenia (Bear, 1982; Bear et al., 1982; Flor-Henry, 1969, 1983; Sherwin, 1977, 2011; Schiff et al., 1982; Stevens et al. 1979; Taylor, 1975). However, this association has also been disputed (see Gold et al. 2014).
Frequently the seizure related disturbances in emotional functioning waxe and wane, such that some patients experience islands of normality followed by islands of psychosis. Possibly these changes are a function in variations in subclinical seizure activity and kindling.
In some instances, the psychiatric disturbance may develop over days as a prelude to the actual onset of a seizure, due presumably to increasing levels of abnormal activity until the seizure is triggered. In other cases, particularly in regard to depression, the alterations in personality and emotionality may occur following the seizure and may persist for weeks or even months (Trimble 2011, 1996).
In yet other instances, patients may act increasingly bizzare for weeks, experience a seizure, and then behave in a normal fashion for some time ("forced normalization", Flor-Henry 1983), only to again begin acting increasingly bizzarre. This has been referred to as an inter-ictal (between seizure) psychosis and is possibly secondary to a build up of abnormal activity in the temporal lobes and limbic system (Bear, 1979; Flor-Henry 1969, 1983) Hence, once the seizure occurs, the level of abnormal activity is decreased and the psychosis goes away only to gradually return as abnormal activity again builds up.
Caveats
Some authors have argued that there is no significant relationship between these psychotic disorders and temporal lobe epilepsy (Gold et al. 2014; Stevens & Hermann, 2011), whereas others have drawn attention to possible social-developmental contributions (Matthews et al., 1982). That is, growing up with a seizure disorder, feeling victimized, not knowing when a seizure may strike, loss of control over ones life, etc. can independently create significant emotional aberrations.
It is also important to emphasize that it is probably only a subpopulation of individuals with temporal lobe epilepsy who come to the attention of most researchers; e.g. those with the most serious or intractable problems. Thus one should not immediately view an individual with temporal lobe (or any type of epilepsy) as immediately suspect for emotional-psychotic abnormalities. Nevertheless, it is noteworthy that not only is there an association between the temporal lobe and certain forms of schizophrenia, but that temporal lobe abnormalities have been noted across generations of individuals and families afflicted with psychotic disturbances (Honer et al. 2014).
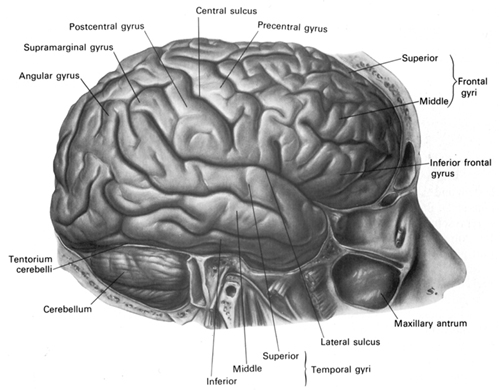
TEMPORAL LOBE INJURY SUSCEPTIBILITY
As detailed in chapters 30, 32, the temporal lobes are highly susceptible to injury from a variety of causes, including head injury, stroke, tumor, and epilepsy. In part, this susceptibility is due to the position of the temporal lobe within the skull. With whiplash injuries, or if the skull is struck from the back or the front, the temporal lobes will slam into the inside of the skull and may be ripped, torn, and sheared. These are called coup and contra coupe injuries.

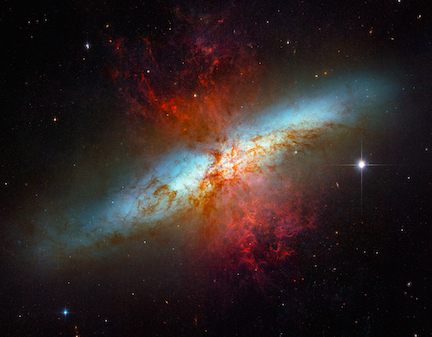
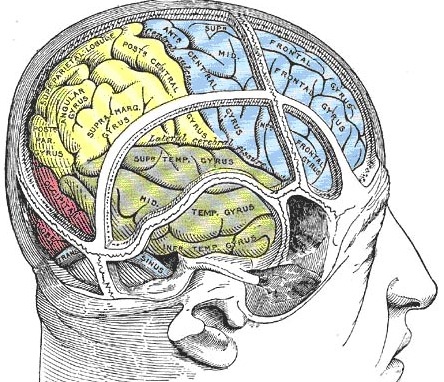
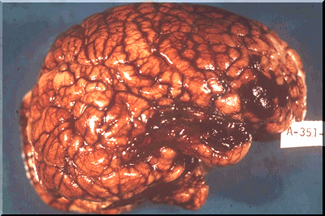
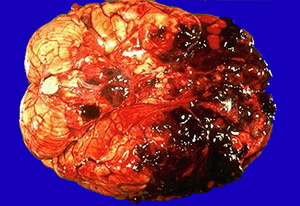
The inferior temporal lobes are also slow to mature which in turn increases the likelihood that abnormal neural networks may be formed in response to adverse early experience (see chapters, 2, 28, 30). Hence, not surprisingly, abnormal early environmental influences, including profound traumatic stress, can induce language, emotional, and memory disorders including repression for childhood experiences, as well as severe psychiatric abnormalities including schizophrenia and dissociative phenomenon; disturbances which implicate the temporal lobes as well as the amygdala and hippocampus.