Rhawn Gabriel Joseph, Ph.D.
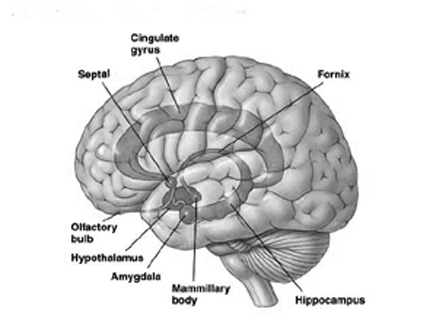
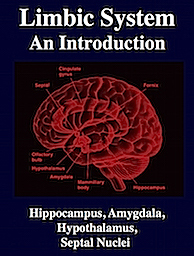
Buried within the depths of the cerebrum are several large aggregates of limbic structures and nuclei which are preeminent in the control and mediation of memory, emotion, learning, dreaming, attention, and arousal, and the perception and expression of emotional, motivational, sexual, and social behavior including the formation of loving attachments. Indeed, the limbic system not only controls the capacity to experience love and sorrow, but it governs and monitors internal homeostasis and basic needs such as hunger and thirst (Bernardis & Bellinger 2007; Gloor 1992, 2010; Joseph, 1990, 1992, 2000a; LeDoux 1992, 2012; MacLean, 1973, 1990; Rolls, 1984, 1992; Smith et al. 1990), including even the cravings for pleasure-inducing drugs (Childress, et al., 2009).
The structures and nuclei of the limbic system are exceedingly ancient, some of which began to evolve over 450 million years ago. Over the course of evolution, these emotional structures have expanded in size, some becoming increasingly cortical in response to increased environmental opportunities and demands. In fact, as the neocortical forebrain expanded and until as recently as 50 million years ago, the cerebrum of the ancestral line that would eventually give rise to humans, was dominated by the limbic system.
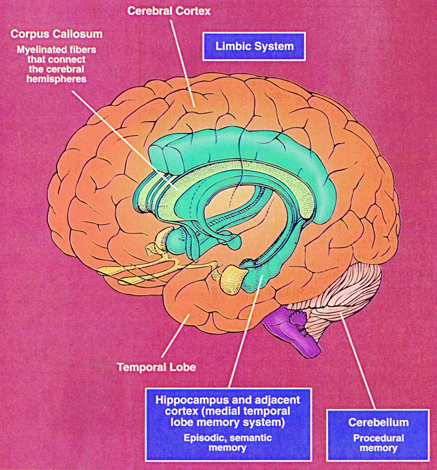
However, over the course of evolution a mantle of neocortex began to develop and enshroud the limbic system; evolving at first to serve limbic needs in a way that would maximize the survival of the organism, and to more efficiently, effectively, and safely satisfy limbic needs and impulses. In consequence, the frontal, temporal, parietal, and occipital lobes evolved covered with a neocortical mantle, that in humans would come to be associated with the conscious, rational mind. Sometimes, however, even in the most rational of humans, emotions can hijack the logical mind, and the neocortex, and even peaceful people might be impelled to murder even those they love.
Indeed, the old limbic brain has not been replaced and is not only predominant in regard to all aspects of motivational and emotional functioning, but is capable of completely overwhelming "the rational mind" due in part to the massive axonal projections of limbic system to the neocortex. Although over the course of evolution a new brain (neocortex) has developed, Homo sapiens sapiens ("the wise may who knows he is wise") remains a creature of emotion. Humans have not completely emerged from the phylogenetic swamps of their original psychic existence.
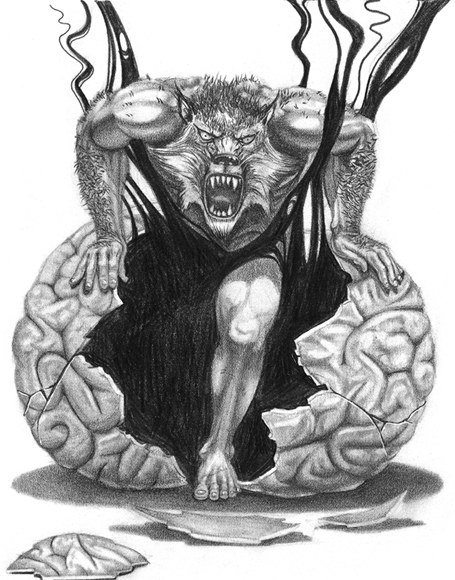
Hence, due to these limbic roots, humans not uncommonly behave "irrationally" or in the "heat of passion," and get into fights, have sex with or scream and yell at strangers thus act at the behest of their immediate desires; sometimes falling "madly in love" and at other times, acting in a blind rage such that even those who are 'loved" may be slaughtered and murdered.
Indeed, emotion is a potentially powerful overwhelming force that warrants and yet resists control-- as something irrational that can happen to a someone ("you make me so angry") and which can temporarily hijack, overwhelm, and snuff out the "rational mind."
The schism between the rational and the emotional is real, and is due to the raw energy of emotion having it's source in the nuclei of the ancient limbic lobe -- a series of structures which first make their phylogenetic appearance over a hundred million years before humans walked upon this earth and which continue to control and direct human behavior.
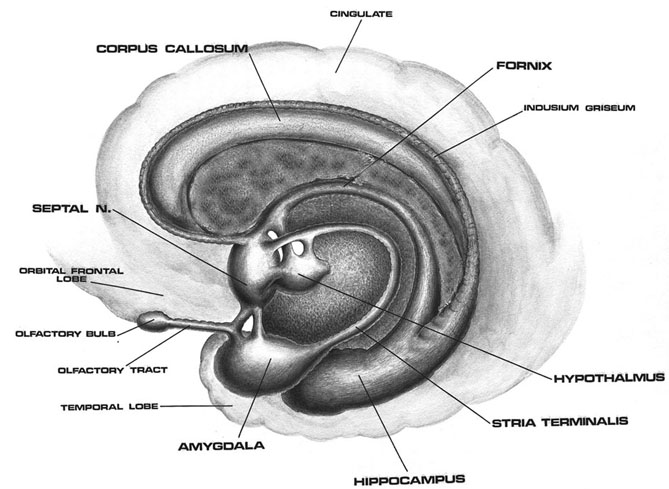
The hypothalamus could be considered the most "primitive" aspect of the limbic system, though in fact the functioning of this sexually dimorphic structure is exceedingly complex. The hypothalamus regulates internal homeostasis including the experience of hunger and thirst, can trigger rudimentary sexual behaviors or generate feelings of extreme rage or pleasure. In conjunction with the pituitary the hypothalamus is a major manufacturer/secretor of hormones and other bodily humors, including those involved in the stress response and feelings of depression.
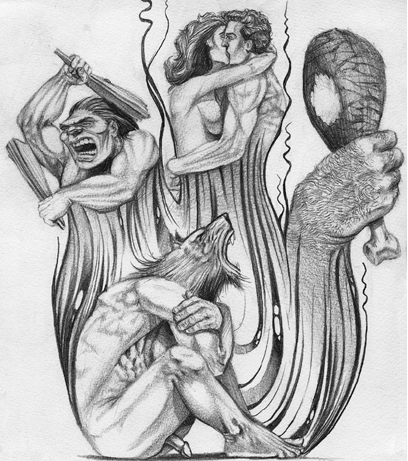
The amygdala has been implicated in the generation of the most rudimentary and the most profound of human emotions, including fear, sexual desire, rage, religious ecstasy, or at a more basic level, determining if something might be good to eat. The amygdala is implicated in the seeking of loving attachments and the formation of long term emotional memories. It contains neurons which become activated in response to the human face, and which become activated in response to the direction of someone else's gaze. The amygdala also acts directly on the hypothalamus via the stria terminalis, medial forebrain bundle, and amygdalafugal pathways, and in this manner can control hypothalamic impulses. The amygdala is also directly connected to the hippocampus, with which it interacts in regard to memory.
The hippocampus is unique in that unlike the amygdala and other structures, almost all of its input from the neocortex is relayed via the overlying entorhinal cortex--a five layered mesocortex. As is well known, the hippocampus is exceedingly important in memory, acting to place various short-term memories into long-term storage. Presumably the hippocampus encodes new information during the storage and consolidation (long-term storage) phase, and assists in the gating of afferent streams of information destined for the neocortex by filtering or suppressing irrelevant sense data which may interfere with memory consolidation. Moreover, it is believed that via the development of long-term potentiation the hippocampus is able to track information as it is stored in the neocortex, and to form conjunctions between synapses and different brain regions which process and store associated memories.

The septal nuclei can produce extremes of emotion, including explosive violence, known as "septal rage."
The septal nuclei is in part an evolutionary and developmental outgrowth of the hippocampus (Ariens Kappers, et al., 1936; Gloor, 2010), and the hypothalamus, and in fact acts to link the hippocampus with the hypothalamus as well as with the brainstem (Andy & Stephan, 1968; Risvold & Swanson, 2012; Swanson & Cowan, 1979;). It consists of both lateral and medial segments; i.e. the lateral and medial septal nuclei (Ariens Kappers, et al., 1936). Presumably, via these interconnections, the septal nuclei exerts modulatory influences on the hippocampus in regard to memory functioning and arousal (Gloor, 2010).
The septal nuclei is also interconnected with and shares a counterbalancing relationship with the amygdala particularly in regard to hypothalamic activity and emotional and sexual arousal (Andy & Stephan, 1968; Swanson & Cowan, 1979). For example, whereas the amygdala promotes indiscriminate contact seeking, and perhaps promiscuous sexual activity, the septal nuclei inhibits these tendencies thus assisting in the formation of selective and more enduring emotional attachments (Joseph, 1992a, 2009b).
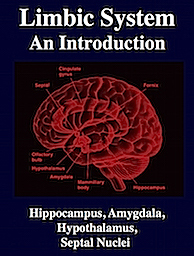
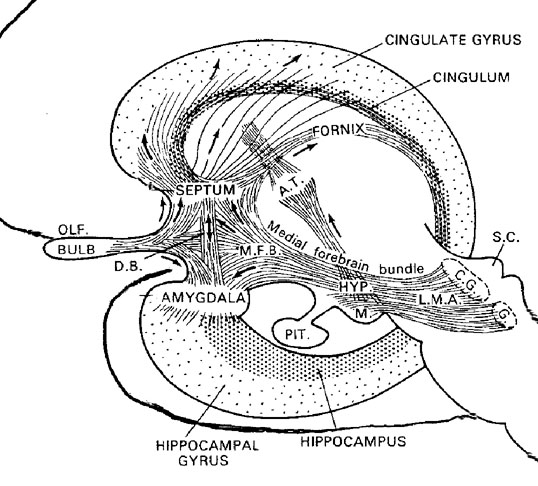
Also implicated in the functioning of the limbic system are the olfactory bulb and olfactory system, the limbic striatum (nucleus accumbens, olfactory tubercle, substantia innominata, ventral caudate and putamen), the orbital frontal and inferior temporal lobes and the midbrain monoamine system. These systems and structures are also directly connected or separated by only a single synapse, and which tend to become aroused not only as a function of emotional arousal, but in reaction to olfactory input which continues to exert profound effects on the human limbic system, and upon human behavior.

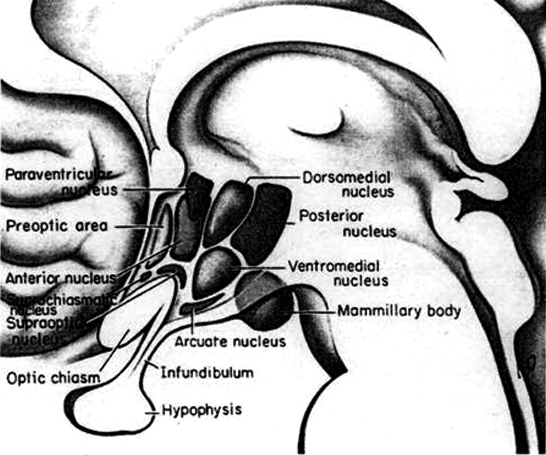
In fact, almost every region of the cerebrum interacts with and communicates with the hypothlamus and is subject to its influences (Swanson, 2007). Moreover, the hypothalamus utilizes the blood supply to transmit hormonal and humoral messages to peripheral organs as well as other brain structures and utilizes the blood supply to receive information as well, thus bypassing the synaptic route utilized by almost all other regions of the neuroaxis (Markakis & Swanson, 2010). Through the blood supply (as well as via the cerebrospinal fluid), the hypothalamus not only regulates, but is subject to feedback regulation by the same structures that it controls.
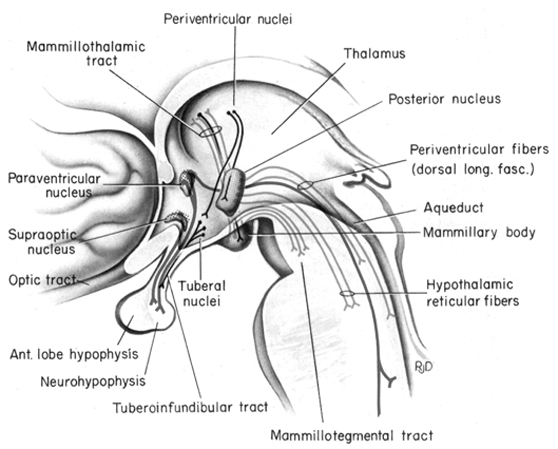
Certain areas of the diencephalon, midbrain, and brainstem, are exceedingly exceedingly sensitive to hormones, humors, and peptides circulating within the blood plasma, and the cerebrospinal fluids; chemosensory information which is used for maintaining homeostasis. Broadly considered, these chemosensory sensitive areas are generally located near or surrounding the cerebral ventricles (Johnson & Gross, 2013) and they tend not to be effected by the so called "blood brain barrier;" referred to as circumventricular organs (CVOs). There are perhaps dozens of CVO's at least 8 of which are located in or near the ventricular systems which feed the brainstem and diencephalon including the hypothalamus, pineal gland and pituitary (Johnson & Gross, 2013).
The hypothalamus, however, does not act solely through the blood supply or via cerebrospinal fluid, and its also receives sensory information synaptically, and often indirectly, as is the case with the majority of olfactory fibers. In general, sensory stimuli reach the hypothalamus from a variety of routes. These include the solitary tract of the brainstem, a structure which receives, processes, and transmits data received principally from the vagus and glosopharyngeal cranial nerves. Through this pathway the lateral hypothalamus is informed about cardivocascular activities, respiration, and taste. These pathways are also bidirectional (Swanson, 2007). Other major pathways include the medial forebrain bundle (which contains axons from a variety of different cellular groups) and the stria terminalis through which the amygdala and hypothalamus interact. The hypothalamus also maintains massive interactive pathways with the frontal lobes and septal nuclei (Risvold & Swanson, 2012).
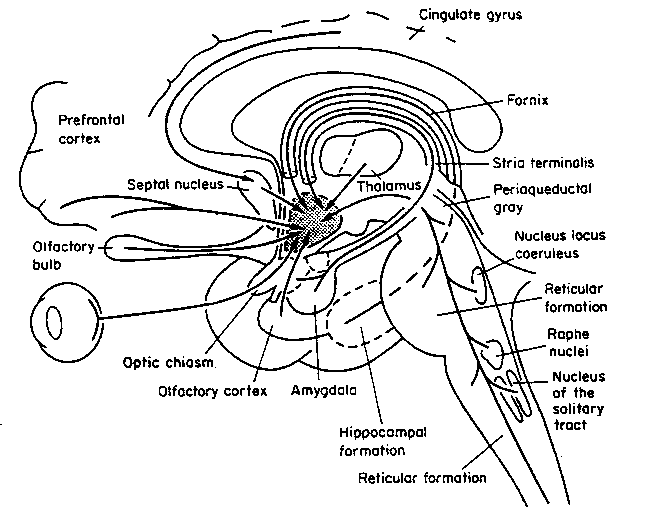
Broadly considered, the hypothalamus consists of three longitudinal subdivisions which extend along its anterior to posterior axis. These are the medial, lateral, and periventricular (Swanson, 2007). The periventricular zone is concerned with neuroendocrine regulation, whereas the lateral and medial zones are concerned with affective states, including hunger and thirst. These zones, in turn can be further subdivided into subnuclei.
Phylogenetically, structurally, and embryologically the hypothalamus is traditionally considered part of the diencephalon. During embryological development it emerges from the diencephalic vessicle of the neural tube along with those anterior-lateral evaginations which become the optic nerves and retina of the eye, as well as the pituitary gland (ventrally) and the pineal gland and thalamus (dorsally). There is some dispute, however, over the developmental patterns of the hypothalamus, as some scientists believe that it develops from the outside in (the "hollow hypothalamus hypothesis").
On the other hand, the hypothalamus originates from the medially situated neuroepithelium, and thus begins its developmental journey in a medial (or rather paramedial) to lateral arc, such that it appears that the medial hypothalamus is fashioned (and matures) in advance of the lateral hypothalamus.
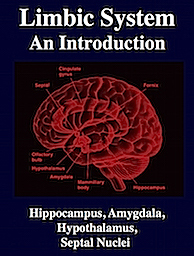
From an evolutionary perspective, however, the hypothalamus appears to have dual (forebrain - midbrain) origins; that is emerging from the dorsal (visual) midbrain, and the olfactory forebrain, which together, and over the course of evolution, gave rise to the ventral, medial, lateral and preoptic hypothalamus. Nevertheless, in modern mammals and humans, the olfactory origins are no longer directly apparent, particularly in that most olfactory fibers reach the hypothalamus indirectly; e.g. via the amygdala and piriform cortex.
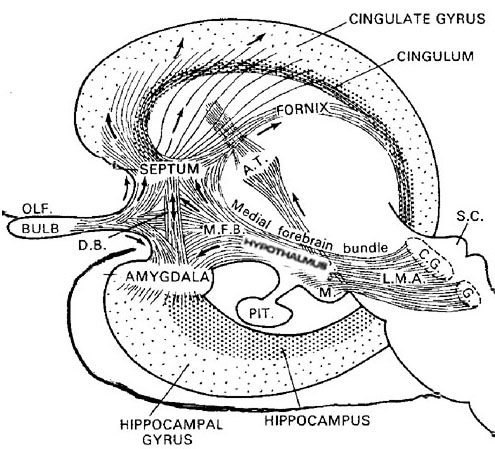
The hypothalamus is exceedingly responsive to olfactory (and pheromonal) input. Perhaps reflecting this partial and putative olfactory origin is the fact that this structure utilizes chemical (hormonal, humoral) molecules to communicate with other areas of the brain, and reacts to these same molecules as well as olfactory cues, including those directly related to sexual status.
It is this olfactory-chemical origin and sensitivity which in turn may explain why portions of the hypothalamus (like the amygdala) are also sexually dimorphic and reacts to pheromonal sensory stimuli including those which signal sexual status. That is, structurally and functionally the hypothalamus of males and females are stucturally dissimilar (Bleier et al. 2002; Dorner, 1976; Gorski et al. 2008; Rainbow et al. 2002; Raisman & Field, 1971, 1973) and perform different functions depending on if one is a man or a woman, and if a woman is sexually receptive, pregnant, or lactating. For example, the sexually dimorphic supraoptic and paraventricular nuclei project (via the infundibular stalk) to the posterior lobe of the pituitary which may then secrete oxytocin--a chemical which can trigger uterine contractions as well as milk production in lactating females (and which can thus make nursing a pleasurable experience). The male hypothalamus/pituitary does not perform this function.
SEXUAL DIMORPHISM IN THE HYPOTHALAMUS
As is well known, sexual differentiation is strongly influenced by the presence or absence of gonadal steriod hormones during certain critical periods of prenatal development in many species including humans. Not only are the external genitalia and other physical features sexually differentiated but certain regions of the brain have also been found to be sexually dimorphic and differentially senstitive to steriods, particularly the preoptic area and ventromedial nucleus of the hypothalamus, as well as the amygdala (Bleier et al. 2002; Dorner, 1976; Gorski et al. 2008; Rainbow et al. 2002; Raisman & Field, 1971, 1973).
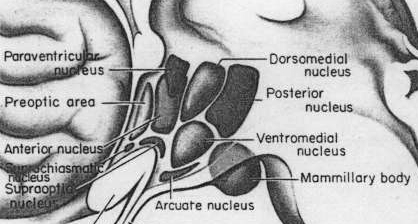
Indeed it has now been well established that the amygdala and the hypothalamus (specifically the anterior commissure, anterior-preoptic, ventromedial and suprachiasmatic nuclei) are sexually differentiated and have sex specific patterns of neuronal and dendritic development, (Allen et al. 1989; Blier et al. 2002; Gorski et al. 2008; Rainbow et al. 2002; Raisman & Field, 1971, 1973; Swaab & fliers, 2005).
This is a consequence of the presence or absence of testosterone during fetal development in humans, or soon after birth in some species such as rodents. Specifically, the presence or absence of the male hormone, testosterone during this critical neonatal period, directly effects and determines the growth and pattern of interconnections between the amygdala and hypothalamus, between axons and dendrites in these nuclei as well as the hippocampus, septal nuclei, olfactory system (ref), and thus the organization of specific neural circuits. In the absence of testosterone, the female pattern of neuronal development occurs. Indeed, it is the presence or absence of testosterone during these early critical periods that appear to be responsible for neurological alterations which greatly effect sex differences in thinking, sexual orientation, aggression, and cognitive functioning (Barnett & Meck, 1990; Beatty, 1992; Dawson et al. 1975; Harris, 2008; Joseph, et al. 2008; Stewart et al. 1975).
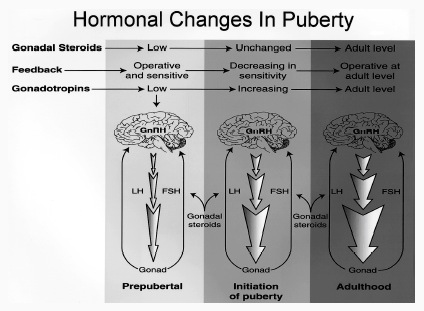
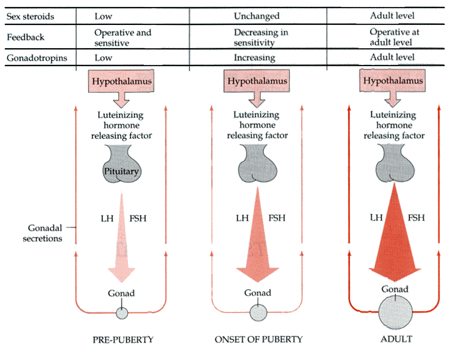
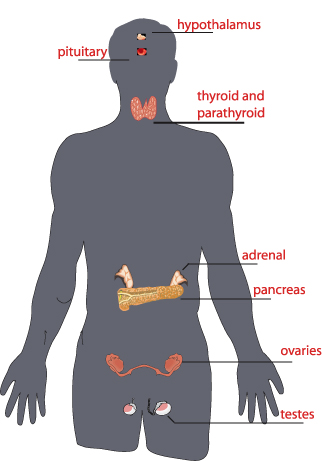
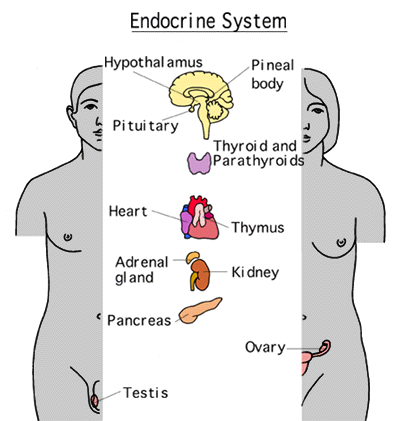
For example, if the testes are removed prior to differentiation, or if a chemical blocker of testosterone is administered thus preventing this hormone from reaching target cells in the limbic system, not only does the female pattern of neuronal development occur, but males so treated behave and process information in a manner similar to females (e.g., Joseph et al. 2008); i.e. they develop female brains and think and behave in a manner similar to females. Conversely, if females are administered testosterone during this critical period, the male pattern of differentiation and behavior results (see Gerall et al. 1992 for review).
That the preoptic and other hypothalamic regions are sexually dimorphic is not surprising in that it has long been known that this area is extremely important in controlling the basal output of gonadotrophins in females prior to ovulation and is heavily involved in mediating cyclic changes in hormone levels (e.g. FSH, LH, estrogen, progesterone). Chemical and electrical stimulation of the preoptic and ventromedial hypothalamic nuclei also triggers sexual behavior and even sexual posturing in females and males (Hart et al., 2005; Lisk, 1967, 1971) and, in female primates, even maternal behavior (Numan, 2005). In fact, dendritic spine density of ventromedial hypothalamic neurons varies across the estrus cycle (Frankfurt et al., 1990) and thus presumably during pregnancy and while nursing.
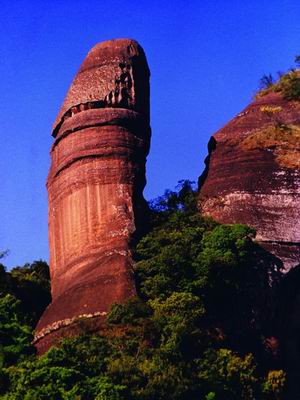
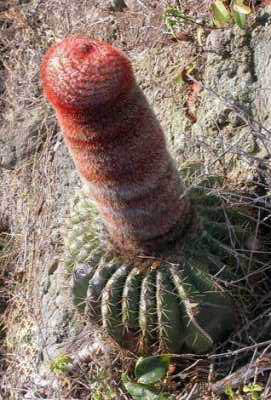
In primates, electrical stimulation of the preoptic area increases sexual behavior in males, and significantly increases the frequency of erections, copulations and ejaculations, we well as pelvic thrusting followed by an explosive discharge of semen even in the absence of a mate (Hart, et al., 2005; Maclean, 1973). Conversely, lesions to the preoptic and posterior hypothalamus eliminates male sexual behavior and results in gonadal atrophy.
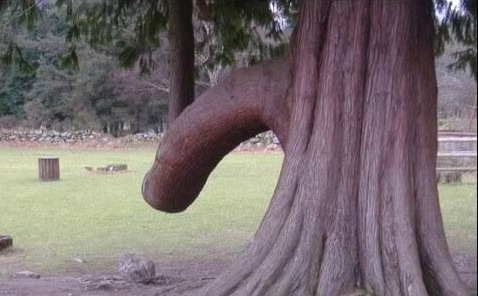
Hence, it is thus rather clear than the ability to sexually reproduce is dependent on the functional integrity of the hypothalamus. In fact, it is via the hypothalamus acting on the pituitary, that gonadotropins come to be released. Gonadotropins control the production and/or release of gametes; i.e. ova and sperm.
Specifically, the hypothalamic neurons secrete gonadotropin-releasing hormone, which acts on the anterior lobe of the pituitary which secretes gonadotropins. However, given that in females, this is a cyclic event, whereas in males sperms are constantly reproduced, is further evidence of the sexual dimorphism of the hypothalamus.
Although the etiology of homosexuality remains in question, it has been shown that the ventromedial and anterior nuclei of the hypothalamus of male homosexuals demonstrate the female pattern of development (Levay, 1991; Swaab, 1990). When coupled with the evidence of male vs female and homosexual differences in the anterior commissure which links the temporal lobe and sexually dimorphic amygdala (see below) as well as the similarity between male homosexuals and women in regard to certain cognitive attributes including spatial-perceptual capability (see below), this raises the possibility that male homosexuals are in possession of limbic system that is more "female" than "male" in functional as well as structural orientation.
It is also interesting to note that the sexually dimorphic preoptic region contains thermosensitive neurons, and controls the physiological and behavior responses to excessive external cold or heat. That is, it is responsible for internal thermoregulation and thus heat loss or retention (Alam et al., 2011). Although we can only speculate, it may well be sex differences in this structure which accounts (at least in part) for the stereotypical differences in male vs female perceptions of cold, and why, stereotypically, females (despite their extra-layers of heat-retaining fat) are more likely to insist on elevating room temperature.
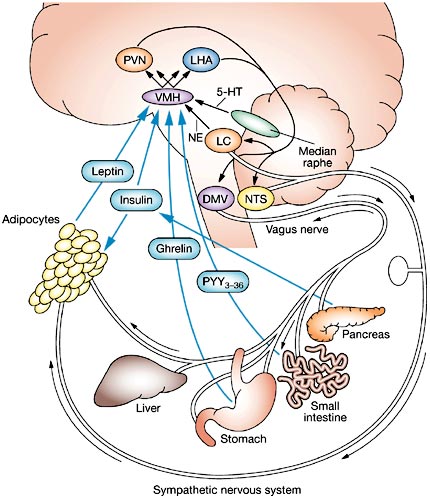
For example, the medial hypothalamus controls parasympathetic activities (e.g. reduction in heart rate, increased peripheral circulation) and exerts a dampening effect on certain forms of emotional/motivational arousal. The lateral hypothalamus mediates sympathetic activity (increasing heart rate, elevation of blood pressure) and is involved in controlling the metabolic and somatic correlates of heightened emotionality (Smith et al. 1990). In this regard, the lateral and medial region act to exert counterbalancing influences on each other.
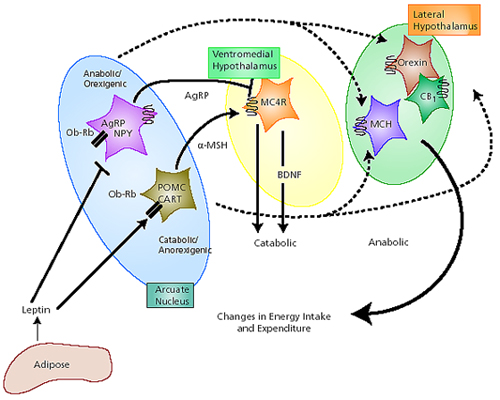
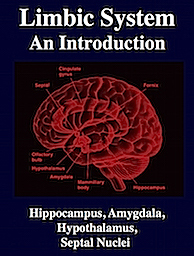
HUNGER & THIRST
The lateral and medial region are highly involved in monitoring internal homeostasis and motivating the organism to respond to internal needs such as hunger and thirst (Anand & Brobeck, 1951; Bernardis & Bellinger 2007; Hetherington & Ranson, 1940). For example, both nuclei appear to contain receptors which are sensitive to the body's fat content (lipostatic/caloric receptors) and to circulating metabolites (e.g. glucose) which together indicate the need for food and nourishment. For example, when food is digested, the viscera secretes various hormones which act on the alimentary tract, which in turn stimulates the solitary tract (ST) which projects directly to the hypothalamus. However, in the absence of food, the viscera also begins to secrete various hormones which when coupled changes in caloric blood levels, signals to the hypothalamus the need for food. The lateral hypothalamus also appears to contain osmoreceptors (Joynt, 1966) which determine if water intake should be altered.
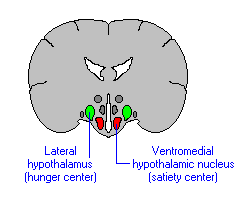
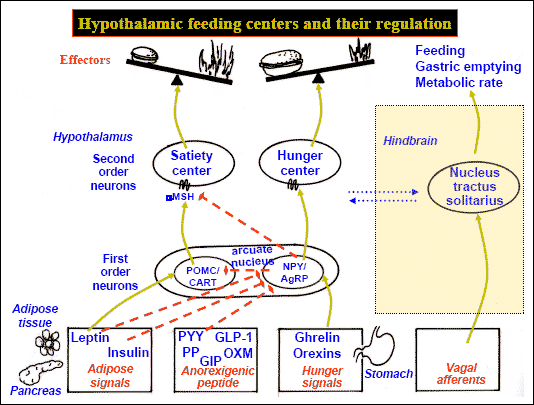
Electophysiologically, it has been determined that the hypothalamus not only become highly active immediately prior to and while the organism is eating or drinking, but the lateral region alters it's activity when the subject is hungry and simply looking at food (Hamburg, 1971; Rolls et. al., 1976). In fact, if the lateral hypothalamus is electrically stimulated a compulsion to eat and drink results (Delgado & Anand, 1953). Conversely, if the lateral area is destroyed bilaterally there results aphagia and adipsia so severe animals will die unless force fed (Anand & Brobeck, 1951; Hetherington & Ranson, 1940; Teitelbaum & Epstein, 1962).
If the medial hypothalamus is surgically destroyed, inhibitory influences on the lateral region appear to be abolished such that hypothalamic hyperphagia and severe obesity result (Anand & Brobeck, 1951; Hoebel & Tetelbaum, 1966; Teitelbaum, 1961). Hence, the medial area seems to act as a satiaty center; but, a center that can be overridden.
Specifically, with ventromedial lesions, animals not only eat more, but the intervals between meals becomes shorter such that they eat more meals. Thus they begin to gain weight. In part this is also due to changes in the sympathetic nervous system which increases vagal activity, thus signaling the need for more food. As noted, the ST is bidirectional.
Normally the hypothalamus can act via the ST and thus the vagal complex and can signal satiation. However, with medial destruction, the ST becomes hyperactive thus inducing parasympathetic overactivity which induces more rapid gastric emptying and the rapid storage of ingested calories. Because these calories are rapidly stored, caloric blood levels are reduced and the lateral hypothalamus is stimulated to begin eating again--which explains the increased frequency of meals. In fact, if animals that have become obese following ventromedial lesions are starved back to their normal weight, once they are allowed free access to food, they again become obese (Hoebel & Tetelbaum, 1966).
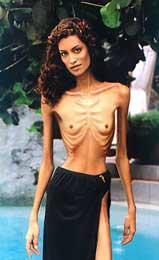
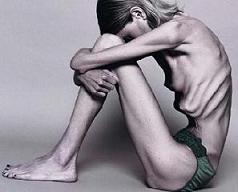
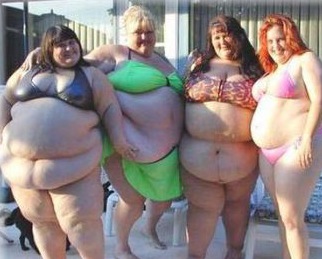
Overall, it appears that the lateral hypothalamus is involved in the intitation of eating and acts to maintain a lower weight limit such that when the limit is reached the organism is stimulated to eat. Conversely, the medial regions seems to be involved in setting a higher weight limit such that when these levels are approached it triggers the cessation of eating.
In part, these nuclei exert these differential influences on eating and drinking via motivational/emotional influences they exert on other brain nuclei (e.g. via reward or punishment). However, it should be stressed that there are a number of other structures and hormones and peptides involved, including the pancreatic islets, and insulin secretion.
PLEASURE & REWARD
In 1952, Heath (cited by Maclean, 1969) reported what was then considered remarkable. Electrical stimulation near the septal nuclei elicited feelings of pleasure in human subjects: "I have a glowing feeling. I feel good!" Subsequently, Olds and Milner (1954) reported that rats would tirelessly perform operants to receive electrical stimulation in this same region and concluded that stimulation "has an effect which is apparently equivalent to that of a conventional primary reward." Even hungry animals would demonstrate a preference for self-stimulation over food.
Feelings of pleasure (as demonstrated via self-stimulation) have been obtained following excitation to a number of diverse limbic areas including the olfactory bulbs, amygdala, hippocampus, cingulate, substantia nigra (a major source of dopamine), locus coeruleus (a major source of norepinephrine), raphe nucleus (serotonin), caudate, putamen, thalamus, reticular formation, medial forebrain bundle, and orbital frontal lobes (Brady, 1960; Lilly, 1960; Olds & Forbes, 1981; Stein & Ray, 2009; Waraczynski et al. 2007).
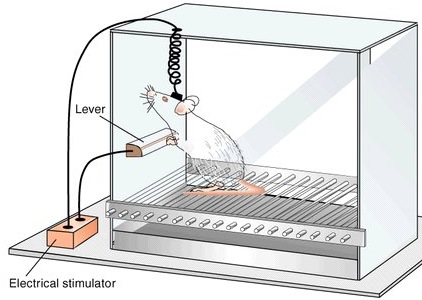
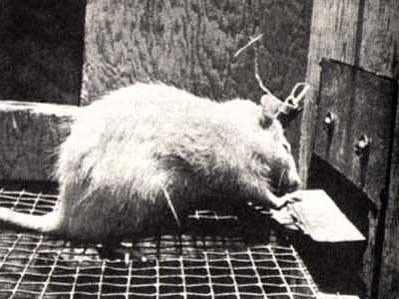
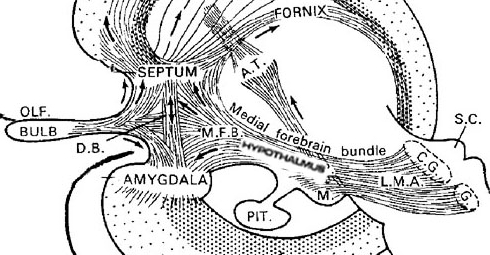
In mapping the brain for positive loci for self-stimulation, Olds (1956) found that the medial forebrain bundle (MFB) was a major pathway which supported this activity. Although the MFB interconnects the hippocampus, hypothalamus, septum, amygdala, orbital frontal lobes (areas which give rise to self-stimulation), Olds discovered in its course up to the lateral hypothalamus reward sites become more densely packed. Moreover, the greatest area of concentration and the highest rates of self-stimulatory activity were found to occur not in the MFB but in the lateral hypothalamus (Olds, 1956; Olds & Forbes, 1981). Indeed, animals "would contine to stimulate as rapidly as possible until physical fatigue forced them to slow or to sleep" (Olds, 1956).
Electrophysiological studies of single lateral hypothalamic neurons indicate that these cells become highly active in response to rewarding food items (Nakamura & Ono, 2006). In fact, many of these cells will become aroused by neutral stimuli repeatedly associated with reward such as a cue-tone --even in the absence of the actual reward (Nakamura & Ono, 2006; Ono et al. 1980). However, this ability to form associations appears to be secondary to amygdaloid activation (Fukuda et al. 2007) which in turn influences hypothalamic functioning.
Nevertheless, if the lateral region is destroyed the experience of pleasure and emotional responsiveness is almost completely attenuated. For example, in primates, faces become blank and expressionless, whereas if the lesion is unilateral, a marked neglect and indifference regarding all sensory events occurring on the contralateral side occurs (Marshall & Teitelbaum, 1974). Animals will in fact cease to eat and will die.
AVERSION
In contrast to the lateral hypothalamus and it's involvement in pleasurable self-stimulation, activation of the medial hypothalamus is apparently so aversive that subjects will work to reduce it (Olds & Forbes, 1981). Hence, electrical stimulation of the medial region leads to behavior which terminates the stimulation--apparently so as to obtain relief (e.g. active avoidance). In this regard, when considering behavior such as eating, it might be postulated that when upper weight limits (or nutritional requirements) are met, the medial region becomes activated which in turn leads to behavior (e.g. cessation of eating) which terminates its activation.
It is possible, however, that medial hypothalamic activity may also lead to a state of quiescence such that the organism is motivated to simply cease to respond or to behave. In some instances this quiescent state may be physiologically neutral, whereas in other situations medial hypothalamic activity may be highly aversive. Quiescence is also associated with parasympathetic activity which is mediated by the medial area.
The hypothalamus, via it's rich interconnections with other limbic regions including the neocortex and frontal lobes, it able to mobilize and motivate the organism to either cease or continue to behave. Nevertheless, at the level of the hypothalamus, the emotional states elicited are very primitive, diffuse, undirected and unrefined.
The organism feels pleasure in general, or aversion/unpleasure in general. Higher order emotional reactions (e.g. desire, love, hate, etc.) require the involvement of other limbic regions as well as neocortical participation.
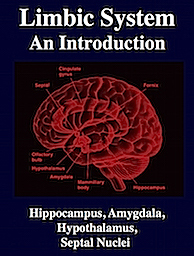
Emotional functioning at the level of the hypothalamus is not only quite limited and primitive, it is also largely reflexive. For example, when induced via stimulation, the moment the electrical stimulus is turned off the emotion elicited is immediately abolished. In contrast, true emotions (which require other limbic interactions) are not simply turned on or off but can last from minutes to hours to days and weeks before completely dissipating.
Nevertheless, in humans, disturbances of hypothalamic functioning (e.g. due to an irritating lesion such as tumor) can give rise to seemingly complex, higher order behavioral-emotional reactions, such as pathological laughter and crying which occurs uncontrollably. However, in some cases when patients are questioned, they may deny having any feelings which correspond to the emotion displayed (Davison & Kelman, 1939; Ironside, 1956; Martin, 1950). In part, these reactions are sometimes due to disinhibitory release of brainstem structures involved in respiration, whereas in other instances the resulting behavior is caused by hypothalamic triggering of other limbic nuclei.
UNCONTROLLED LAUGHTER
Pathological laughter has frequently been reported to occur with hypophyseal and midline tumors involving the hypothalamus, aneurysm in this vicinity, hemorrhage, astrocytoma or pappiloma of the 3rd ventricle (resulting in hypothalamic compression), as well as surgical manipulation of this nucleus (Davison & Kelman, 1939; Dott, 1938; Foerster & Gabel, 1933; Martin, 1950; Money & Hosta, 1967; Ironside, 1956; List, Dowman, & Bagheiv, 1958).
For example, Martin (1950, p.455) describes a man who while "attending his mother's funeral was seized at the graveside with an attack of uncontrollable laughter which embarrassed and distressed him considerably." Although this particular attack dissipated, it was soon accompanied by several further fits of laughter and he died soon thereafter. Post-mortem a large ruptured aneurysm was found, compressing the mammillary bodies and hypothalamus.
In a similar case (Anderson, 1936; Cited by Martin, 1950), a patient literally died laughing following the eruption of the posterior communicating artery which resulted in compression (via hemorrhage) of the hypothalamus. "She was shaken by laughter and could not stop: short expirations followed each other in spasms, without the patient being able to make an adequate inspiration of air, she became cyanosed and nothing could stop the spasm of laughter which eventually became noiseless and little more than a grimace. After 24 hours of profound coma she died."
Because laughter in these instances has not been accompanied by corresponding feeling states, this pseudo-emotional condition has been referred to as "sham mirth" (Martin, 1950). However, in some cases, abnormal stimulation in this region (such as due to compression effects from neoplasm) has triggered corresponding emotions and behaviors -- presumably due to activation of other limbic nuclei.
For example, laughter has been noted to occur with hilarious or obscene speech--usually as a prelude to stupor or death--in cases where tumor has infiltrated the hypothlamus (Ironside, 1956). In several instances it has been reported by one group of neurosurgeons (Foerster & Gagel, 1933) that while swabbing the blood from the floor of the 3rd ventricle, patients "became lively, talkative, joking, and whistling each time the infundibular region of the hypothalamus was manipulated." In one case, the patient became excited and began to sing.
HYPOTHALAMIC RAGE
Stimulation of the lateral hypothalamus can induce extremes in emotionality, including intense attacks of rage accompanied by biting and attack upon any moving object (Flynn et al. 1971; Gunne & Lewander, 1966; Wasman & Flynn, 1962). If this nucleus is destroyed, aggressive and attack behavior is abolished (Karli & Vergness, 1969). Hence, the lateral hypothalamus is responsible for rage and aggressive behavior.
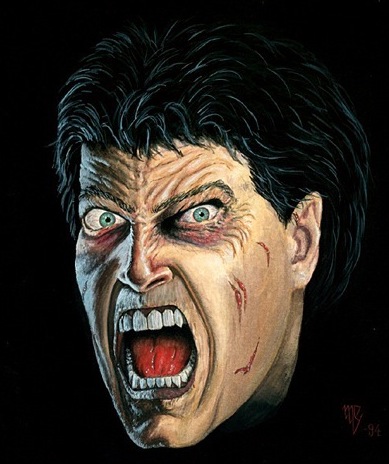
As noted, the lateral maintains an oppositional relationship with the medial hypothalamus. Hence, stimulation of the medial region counters the lateral area such that rage reactions are reduced or eliminated (Ingram, 1952; Wheately, 1944), whereas if the medial is destroyed there results lateral hypothalamic release and the triggering of extreme savagery.
In man, inflammation, neoplasm, and compression of the hypothalamus have also been noted to give rise to rage attacks (Pilleri & Poeck, 1965), and surgical manipulations or tumors within the hypothalamus have been observed to elicit manic and rage-like outbursts (Alpers, 1940). These appear to be release phenomenon, however. That is, rage, attack, aggressive, and related behaviors associated with the hypothalamus appears to be under the inhibitory influence of higher order limbic nuclei such as the amygdala and septum (Siegel & Skog, 1970). When the controlling pathways between these areas are damaged (i.e. disconnection) sometimes these behaviors are elicited.
For example, Pilleri and Poeck (1965) described a man with severe damage throughout the cerebrum including the amygdala, hippocampus, cingulate, but with complete sparing of the hypothalamus who continually reacted with howling, growling, and baring of teeth in response to noise, a slight touch, or if approached. Hence, the hypothalamus being released responds reflexively in an aggressive-like non-specific manner to any stimulus. Lesions of the frontal-hypothalamic pathways have been noted to result in severe rage reactions as well (Fulton & Ingraham, 1929; Kennard, 1945).
Nevertheless, like "sham mirth", rage reactions elicited in response to direct electrical activation of the hypothalamus immediately and completely dissipate when the stimulation is removed. As such, these outbursts have been referred to as "sham rage".
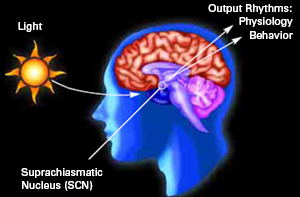
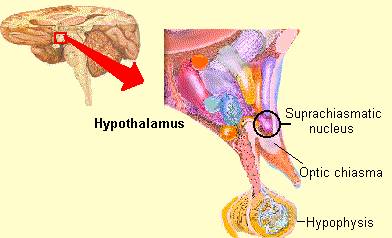
In humans and other species, the SCN (and the midbrain superior colliculus) is a direct recipient of retinal axons. It also receives indirect visual projections from the lateral geniculate nucleus of the thalamus (see Morin 2014). In this regard, the visual system appears to act to synchronize the SCN (and probably the midbrain-pons) to function in accordance with seasonal and day to day variations in the light/dark ratio. However, the SCN does not "see" per se, nor can it detect visual features, as its main concern is adjusting mood, and activity in regard to light intensity as related to rhythm generation.
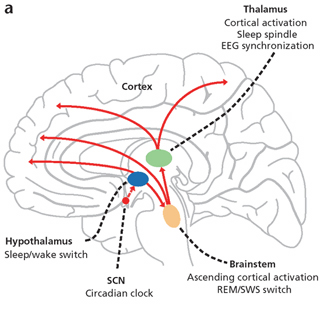
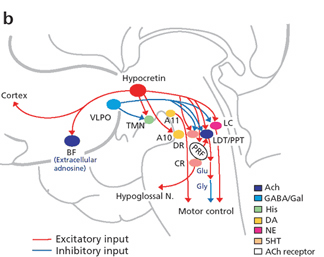
There is thus some evidence which suggests that when the SCN of the hypothalamus is deprived of (or unable to effectively respond to) sufficient light, although rhythm generation is not grossly effected (Morin 2014), individuals may become depressed; a condition referred to as Seasonal Affective Disorder (SAD). That is, the hypothalamus (and midbrain-pons) appear to decrease those hormonal and neurochemical activities normally associated with activation and high (daytime) activity thus resulting in depression.
For example, the hypothalamic-pituitary axis secretes melatonin in phase with the circadian rhythm. Phase-delayed rhythms in plasma melatonin secretion have been repeatedly noted in most (but not all) studies of individuals with SADs (see Wirz-Justice et al. 2013, for review). However, with light therapy, not only is the depression relieved but the melatonin secretions return to normal. This is significant for melatonin is derived from tryptophan via serotonin and low serotonin levels have been directly linked to depression (e.g. Van Pragg 2002).
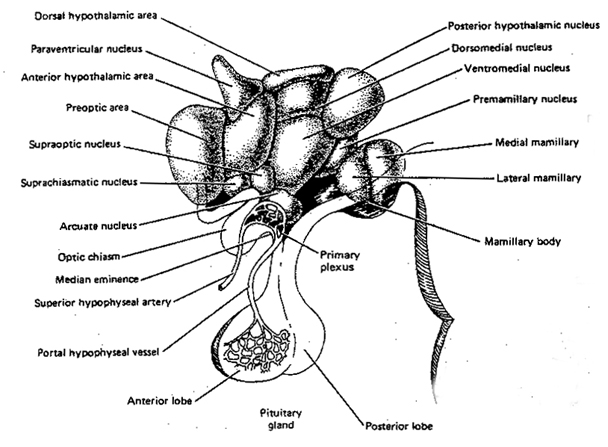
There is some evidence which suggests that the hypothalamus (and the midbrain) may act to regulate serotonin release within the brainstem (Chaouloff 2013; however, see Morin 2014), which in turn may explain why serotonin levels rhythmically fluctuate (e.g. such as during the sleep cycle), or become abnormal when denied sufficient light; i.e. the production of serotonin by the raphe nucleus (in the pons) is abnormally effected.
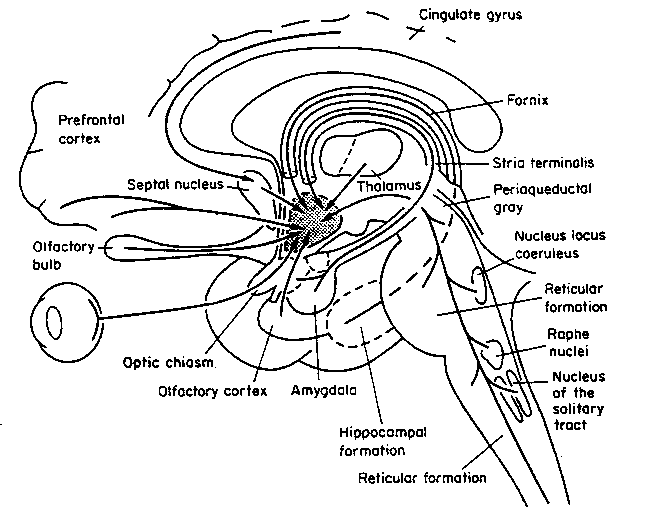
On the other hand, numerous studies have reported that SADs and major depression occurs most often during the Spring and not the winter, and is not influenced by latitude (e.g. Margnusson & Stefansson 2013; Wirz-Justice et al. 2013). There is also some suggestion that abnormal temperature perception, or aging within the SCN may be responsible for the genesis of SADs and related depressive disorders. For example, age related changes in the SCN have been noted to adversely effect circadian rhythm generation as well as metabolic and peptide activity (Aronson et al. 2013). In consequence, rest vs active cycles also become abnormal, with reductions in arousal and activity; i.e. the patient becomes depressed.
It is also possible, however, that although light therapy can assist in alleviating depressive symptoms associated with SADs, that the deregulation of the SCN (and melatonin/serotonin) might be unrelated to light, temperature, or aging, but may be a consequence of stress on the hypothalamus (Chauloff 2013). For example, the hypothalamic-pituitary axis is tightly linked with and in fact mediates stress induced alterations in serotonin (see Chauloff 2013, for review); as well as norepinephrine (Swann et al. 2014) which has also been repeatedly implicated in the genesis of depression.
These events in turn appear to be under the modulating influences of norepinephrine. That is, as stress increases, NE levels decrease, which triggers the activation of the HPA axis. As is well known, low levels of NE are associated with depression.
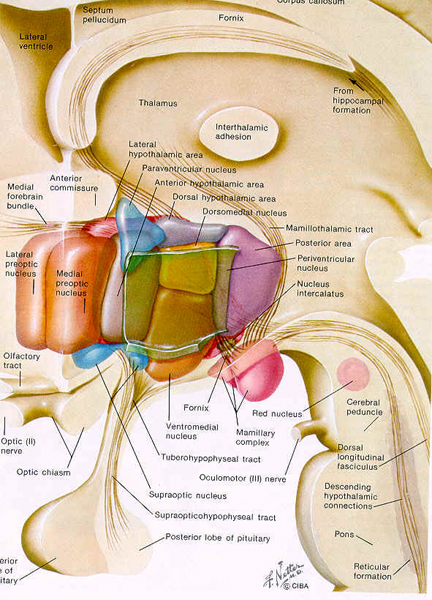
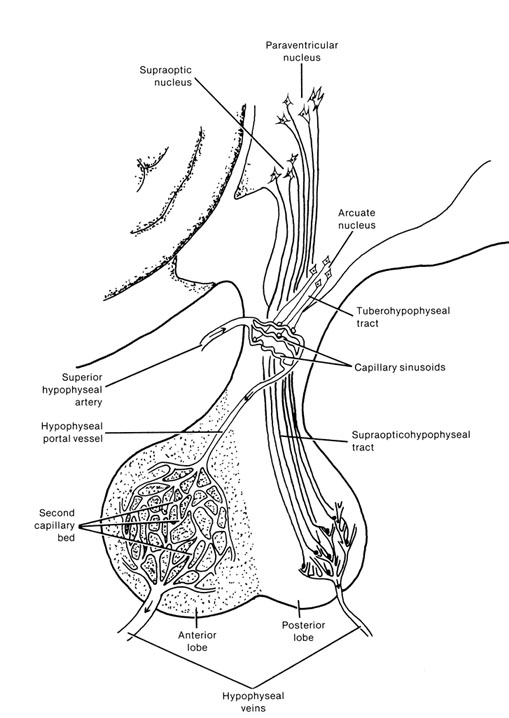
Normally, cortisol secretion is subject to the tonic influences of NE; whereas cortisol can indirectly reduce NE synthesis. Thus a feedback system is maintained via the interaction of these substances (in conjuction with ACTH). Moreover, cortisol and NE levels fluctuate in reverse, and thus maintain a reciprocal relationship with the circadian rhythm; i.e. in oppositional fashion they increase and then decrease throughout the day and evening.
Among certain subgroups suffering from depression, it appears that this entire feedback regulatory system and thus the HPA axis is disrupted (Carrol et al. 1976; Sachar et al. 1973). This results in the hypersecretion of ACTH and cortisol with a corresponding decrease in NE; which results in NE induced depression. It was these findings which led to the development of the Dexamethasone suppression test over 25 years ago.
Via the administration of Dexamethasone (a synthetic corticosteroid) it was determined that many depressed individuals have excess cortisol, and an increased frequency of cortisol secretory episodes (Carrol et al. 1976; Sachar et al. 1973; Swann et al. 2014). Moreover, those who demonstrate excess cortisol were found to respond to NE potentiating agents, whereas those who were depressed but with normal cortisol, responded best to serotonin potentiating compounds (Van Pragg 2002).
It is also noteworthy that dexamethason nonsuppression rates are increased in mania; specifically "mixed manic" states which consist of lability, grandiosity, and lability superimposed over depression (see Swann et al. 2014). These "mixed manic" individuals also display elevated NE levels but respond poorly to lithium and show higher levels of cortisol during the depressed phase of their illness (Swann et al. 2014).
As noted, the hypothalamus may greatly influence circadian activities within the midbrain and pons, and thus the rhythmical secretion of various neurotransmitters. For example, corticotropin-releasing factor acts directly on the locus coeruleus (Valentino et al. 1983) which manufactures NE, and on the raphe thereby influencing serotonin release. These findings suggest a disturbance in circadian or rhythmical control of hypothalamic and midbrain-pontine activity can give rise to depression, or mixed mania in some individuals; women in particular.
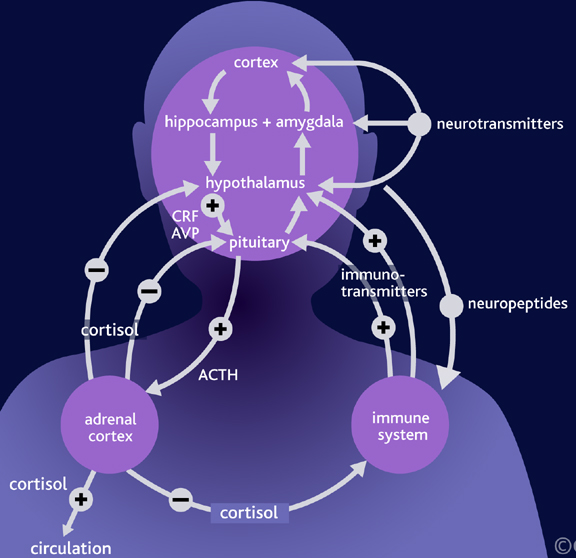
Lateralization.
Although scant, there is some evidence which suggests that the right hypothalamus may be more heavily involved in the control of neuroendocrine functioning, particularly in females. Females are also far more likelyt to suffer from depression and from SADs. Moreover, right cerebral dysfunction can reduce NE levels in both the right and left hemisphere (Robinson 1979). Greater right hypothalamic concentration of substances such as LHRH (luteinizing hormone) has also been reported (Gerendai, 1984), which in turn is a "female" hormone involved in lactation and pregnancy.
Biologically the hypothalamus serves the body tissues by attempting to maintain internal homeostasis and by providing for the immediate discharge of tenions in an almost reflexive manner. Hence, as based on studies of lateral and medial hypothalamic functioning, it appears to act reflexively, in an almost on/off manner so as to seek or maintain the experience of pleasure and escape or avoid unpleasant, noxious conditions.
Emotions elicited by the hypothalamus are largely undirected, short-lived, being triggered reflexively and without concern or understanding regarding consequences; that is, unless chronically stressed or aroused. Nevertheless, direct contact with the real world is quite limited and almost entirely indirect as the hypothalamus is largely concerned with the internal environment of the organism. Although it receives and responds to light, it cannot "see." It has no sense of morals, danger, values, logic, etc., and cannot feel or express love or hate. Although quite powerful, hypothalamic emotions are largely undifferentiated, consisting of feelings such as pleasure, unpleasure, aversion, rage, hunger, thirst, etc.
As the hypothalamus is concerned with the internal enviornment much of it's activity occurs outside conscious-awareness. Moreover, being involved in maintaining internal homeostasis, via, for example, it's ability to reward or punish the organism with feelings of pleasure or aversion, it tends to serve what Freud (1911) has described as the pleasure principle.
THE PLEASURE PRINCIPLE
The lateral and medial nuclei exert counterbalancing influences which serve to modulate activity occurring in the other. As described by Freud (1911), the pleasure principale not only serves to maximize pleasant experiences, but acts to keep the psyche as a whole free from high levels of excitation (be they pleasurable or unpleasant).
Like the hypothalamus, the pleasure principle is present from birth and for some time thereafter the search for pleasure is manifested in an unrestricted manner and with a great deal of intensity as there are no oppositional forces (except those between the lateral and medial regions) to counter it's strivings. Indeed, higher order limbic nuclei have yet to mature.
Functionally isolated, the hypothalamus at birth has no way of reducing tension or mobilizing the organism for any form of effective action. It is helpless. When tensions associated with immediate needs (e.g. hunger or thirst) become unpleasant the only response available to the hypothalamus is to cry and make rage-like vocalizations. When satiated, the hypothalamus can only respond with a feeling state suggesting pleasure or at least quiescence. Indeed, as is well known, for the first few months of life the infants awareness largely consists of a very restricted matrix involving tactile, visceral (hunger) and kinesthetic sensations, where emotionally the infant is capable of screaming, crying, or demonstrating very rudimentary features of pleasure, i.e. an attitude of acceptance of quiescence (McGraw, 1969; Milner, 1967; Piaget, 1952; Spitz & Wolf, 1946).
It is only with the further differentiation and maturation of higher order limbic nuclei (e.g. amygdala, septal nucleus, hippocampus) that the infant begins to achieve some awareness of external reality and begins to form memories as well as differentiate and associate externally occurring events and individuals.
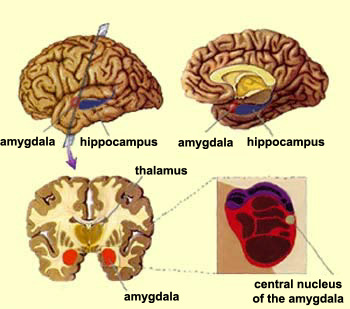
In contrast to the primitive hypothalamus, the more recently developed amygdala (the "almond") is preeminent in the control and mediation of all higher order emotional and motivational activities. Via it's rich interconnections with various neocortical and subcortical regions, amygdaloid neurons are able to monitor and abstract from the sensory array stimuli that are of motivational significance to the organism (Gaffan 1992; Gloor 1960, 1992, 2010; LeDoux 1992; Morris et al., 2012; Rolls, 1984, 1992 Steklis & Kling, 2005; Kling & Brothers 1992; Ursin & Kaada 1960). This includes the ability to discern and express even subtle social-emotional nuances such as friendliness, fear, love, affection, distruct, anger, etc., and at a more basic level, determine if something might be good to eat.
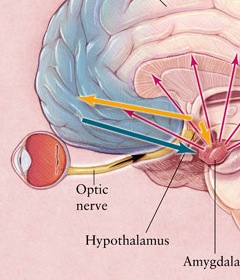
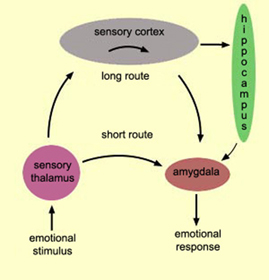
In fact, amygdaloid neurons respond selectively to the flavor of certain preferred foods, as well as to the sight or sound of something that might be especially desirable to eat (Fukuda et al. 2007; Gaffan et al. 1992; O'Keefe & Bouma, 1969; Ono et al. 1980; Ono & Nishijo, 1992) including even the sight of drugs that induce extreme pleasure.
For example, it has been shown, using positron emission tomography, that detoxified cocaine users not only respond to a cocaine video with cocaine craving, but with increased amygdala (and anterior cingulate) activity (Childress, et al., 2009).
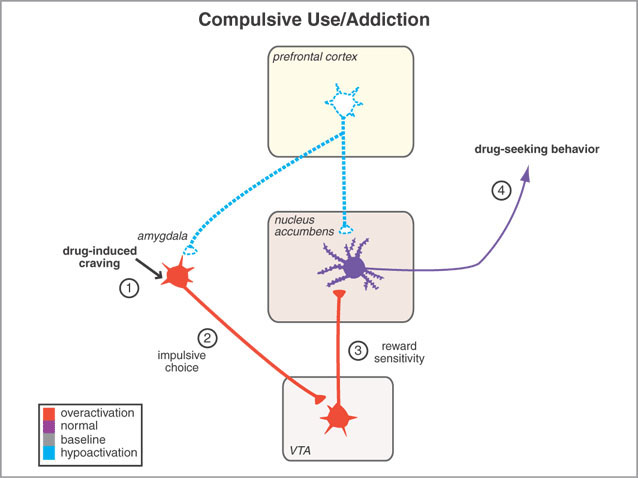
Belying its involvement in emotion, including the pleasure associated with cocaine usage, is the unique chemical anatomy of the amygdala, which is rich in a variety of neuropetides including enkephalins and beta-endorphins as well as opiate receptors (Atweh & Kuhar, 1977; Fallon & Ciofi, 1992; Uhl et al. 2008). In fact, of all brain regions, the greates concentration of opiate receptors is found within the human amygdala. Other chemical systems include lutenizing hormone, vasopressin, somatostatin, and corticotropin releasing factor (Fallon & Ciofi, 1992) --indications of its involvement in stress and sexuality, especially female sexuality. The primate amygdala is sexually differentiated with male and female patterns of dendritic organization and steroid activity (Bubenik & Brown, 1973; Nishizuka & Arai, 1981; see also Simerly, 1990).
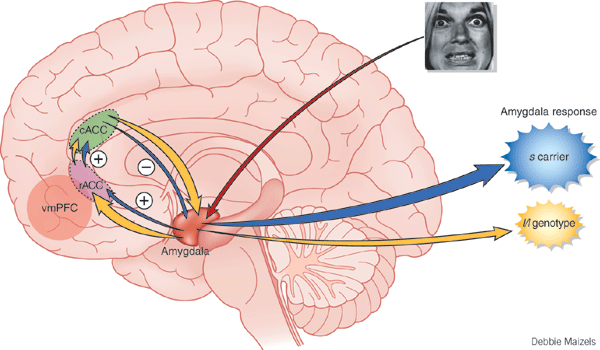
The amygdala is exceedingly responsive to social and emotional stimuli as conveyed vocally, through touch, sight, and via the expressions of the face (Gloor, 1992; Halgren, 1992; Kling & Brothers 1992; Morris et al., 2012; Rolls, 1984, 1992). In fact, the amygdala, as well as the overlying (and partly coextensive) temporal lobe, contains neurons which respond selectively to smiles and to the eyes, and which can differentiate between male and female faces and the emotions they convey (Hasselmo, Rolls, & Baylis, 1989, Heit et al., 1988; Kawashima, et al., 2009; Rolls, 1984). For example, the left amygdala acts to discriminate the direction of another person's gaze, whereas the right amygdala becomes activated while making eye-to-eye contact (Kawashima, et al., 2009).
Moreover, the normal human amygdala typically responds to frightened faces by altering its activity (Morris et al., 2012), whereas injury to the amygdala disrupts the ability to recognize faces (Young, Aggleton, & Hellawell,2011). With bilateral destruction, emotional speech production and the capacity to respond appropriately to social emotionally stimuli is abolished (Lilly, Cummings, Benson, & Frankel, 1983; LeDoux, 2012; Marlowe, Mancall, Thomas,1975; Scott, Young, Calder, Hellawell, Aggleton, & Johnson, 2010; Terzian & Ore, 1955).
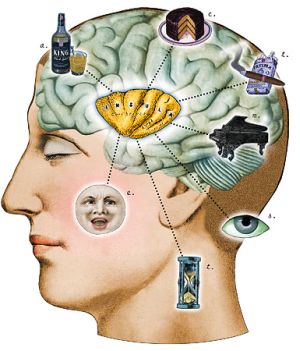
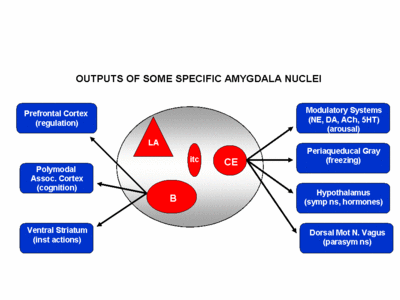
Single amygdaloid neurons receive a considerable degree of topographic input, and are predominatly polymodal, responding to a variety of stimuli from different modalities simultaneously (Amaral et al. 1992; O'Keefe & Bouma, 1969; Ono & Nishijo, 1992; Perryman, Kling, & Lloyd, 2007; Rolls 1992; Sawa & Delgado, 1963; Schutze et al. 2007; Turner et al. 1980; Ursin & Kaasa, 1960; Van Hoesen, 1981). The amygdala is also very sensitive to somesthetic input and physical contact such that even a slight touch in a very circumscribed area of the body can produce amygdaloid excitation. Overall, because emotional, motivational, and multimodal assimilation of various sensory impressions occurs in this region, it is also involved in attention, learning, and memory (Gloor, 2010; Halgren, 1992; LeDoux, 2012).
Moreover, through the massive interconnections maintained with the lateral and medial (ventromedial) hypothalamus, the amygdala is able to act directly on this structure, driving the hypothalamus, so to speak, and thus tapping into its emotional reserviour so that its ends may be met. Indeed, it is able to modulate hypothalamic activity through inhibitory and excitatory projections to this structure (Dreifuss, et al., 1968).
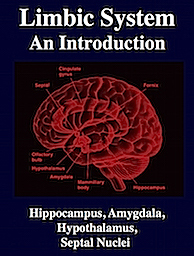
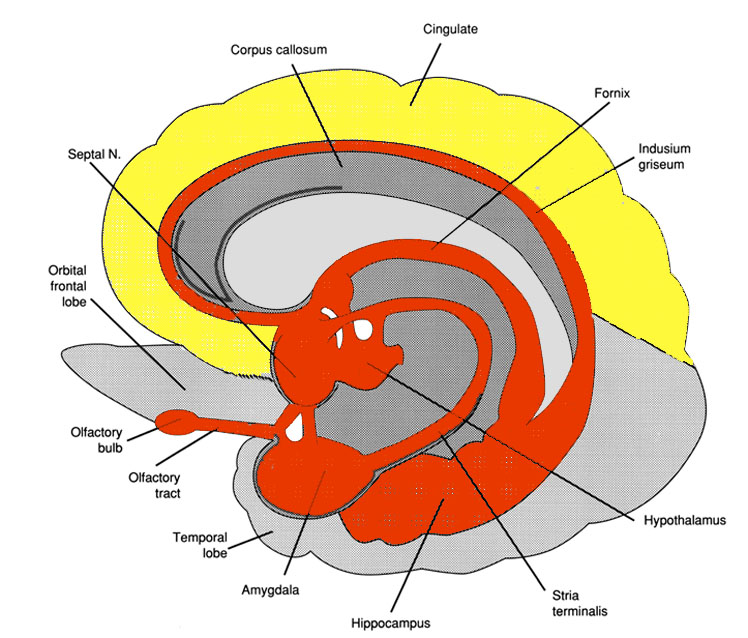
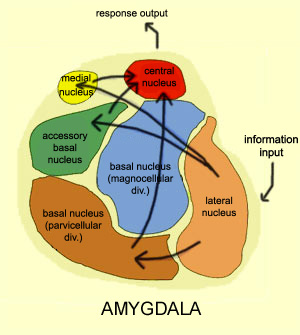
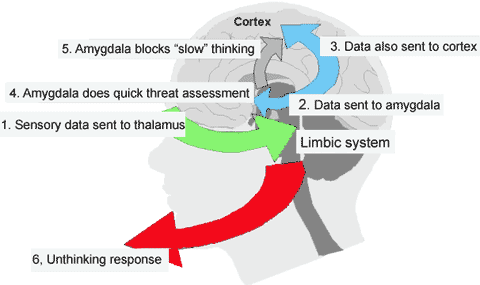
Direct stimulation of the basolateral amygdala and the ventral amydalofugal pathway excites the principle neurons of the medial hypothalamus (Dreifuss, et al., 1968). By contrast, stimulation of the medial (ventro-medial) amygdala and the stria terminalis pathway, inhibits these same hypothalamic neurons (Dreifuss, et al., 1968). Hence, whereas the lateral amydala exerts excitatory influences on the hypothalamus, the medial amygdala exerts inhibitory influences, and can thus control, or at least exert excitatory/inhibitory and thus modulatory influences on hunger, thirst, sexual arousal, rage, etc., as well as hormonal, endocrine, and other functions associated with the hypothalamic nuclues (Dreifuss, et al., 1968; Joseph, 1992a; Gloor, 2010). Indeed, the amygdala can be likened to the chief executive of the limbic system and weilds enormous power via its control over the hypothalamus.
For example, in the cat and monkey, stimulation of the border area between the lateral and medial hypothalamus can trigger aggressive defensive reactions (De vito & Smith, 2002; Hess, 1949). As indicated by radioactive tracers, both the lateral and medial amygdala projection to this area (De vito & Smith, 2002). And, when the amygdala is electrically activated, the hypothalamus becomes activated (Dreifuss, et al., 1968), and defensive and aggressive reactions can be triggered.
However, this system is also interactional, especially in regard to sexual activity, fear, anger, hunger, and stress. For example, the hypothalamus can stimulate the amygdala which may then survey the environment so that internal needs may be met, and/or they may act in concert regarding sexual behavior, the stress response, and so on.
OVERVIEW: AMYGDALA STRUCTURAL FUNCTIONAL ORGANIZATION
The amygdala is buried within the depths of the anterior-inferior temporal lobe and consists of several major nuclear groups including what has been referred to as the "extended amygdala." These include the cortical-medial, central, paralaminar, lateral, basal, and acessory basal nucleus (Amaral et al., 1992; Stephan & Andy, 1977). Different authors propose different divisions and link them differently. For example, Stephan and Andy (1977) assign the cortical division to the basolateral amydala, and the central division to the medial division. Price et al., (1977) subdivided the amygdala into basolateral, corticomedial and central amygdaloid nuclei. Others propose yet different schemes.
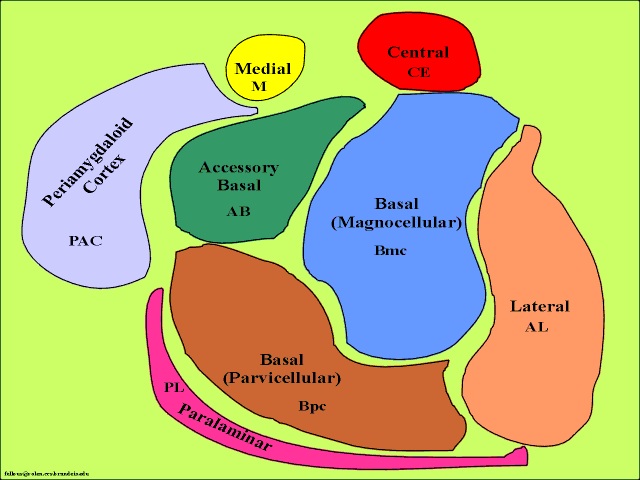
For our purposes we will primarily focus on the "medial" and the basolateral subdivisions. The phylogenetically ancient medial group (or cortico-medial amygdala) is involved in olfaction, sexual, and motor activity (via its interconnections with the striatum), and the relatively newer basolateral division (lateral amygdala) is most fully developed in primates and humans (Amaral et al. 1992; Herrick, 1925; Humphrey, 1972; McDonald 1992; Stephan & Andy, 1977). Of its various subdivisions, the basolateral amygdala is the most "cortex-like. However, being allocortex it contains three layers (vs 7 for the neocortex) with layer 2 containing pyramidal neurons which rely on excitatory neurotransmitters, e.g., glutamate--whereas the local-circuit (interneurons) rely on the inhibitory transmitters, e.g., GABA (Fallon & Ciofi, 1992).
The lateral amydala utilizes the ventral amygdalofugal pathway and less so the stria terminalis to influence the hypothalamus. The lateral amygdala also relies on the medial forebrain bundle which is the pathway subserving the pleasure circuit (Olds & Forbes, 1981). By contrast, the medial amygdala relies on the stria terminalis, and less so the amygdalofugal pathway to influences the hypothalamus. Through these pathways, these diferent subdivions of the amygdala can act to modulate activity in the hypothalamus, septal nuclei, and other subcortical structures (Amaral et al. 1992; Stephan & Andy, 1977).
Evolution & Embryology
In humans, the right amygdala is also larger than the left amygdala, with the basolateral portion contributing to most of this asymmetry (Murphy et al., 2007). Moreover, over the course of evolution, and in the transition from amphibians to reptiles, to mammals and then humans, the basolateral amygdala appears to have grown the most in size as compared to other amygdaloid nuclei (Stephan & Andy, 1977)--that is, when considered only in regard to its subtemporal diminsions. However, it also appears that the medial amygdala may have contributed to the evolution of the piriform cortex, and then the evolution of the 4 to 5 layered mesocortex, which when layered upon the 3-layered allocortical piriform cortex, gave rise to the neocortex of the temporal lobe. Hence, the expansion of the medial amydala may not be apparent as that expansion is represented as mesocortex and neocortex.
Moreover, it appears that the medial group was broken up over the course of evolution such that structures such as the claustrum (Gilles et al., 1983), became separated and is now situated beneath the auditory cortex of the superior temporal lobe. Indeed, the claustrum which is very cortical in organization, may well act as an interface between the auditory cortex and the amygdala, processing and relaying auditory impulses to and fro, and may have, in addition to the medial amygdala, contributed to the evolution of the auditory cortex.
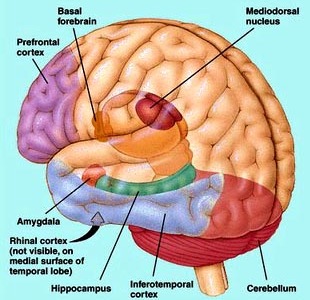
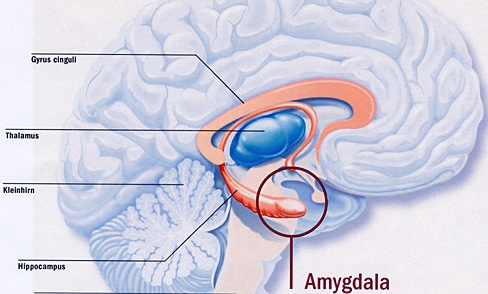
The amygdala, therefore, has definitely increased in size over the course of evolution (Stephan & Andy, 1977), and has become increasingly cortical, has contributed to the evolution of mesocortex and neocortex (which is thus a cortical extension of the neocortex, maintaining extensive interconnections). In addition, the right amygdala is larger than the left (Murphy et al., 2007) which in turn may contribute to right hemisphere dominance for emotion (chapter 10).
Intrinsic & Extrinsic Organization: The Flow of Information
Like the lateral and medial hypothalamus, the medial and basolateral (hereafter referred to as the lateral) amygdaloid nuclei subserve different functions and maintain different anatomical interconnections (Amaral et al., 1992; Stephan & Andy, 1977). And, they can be subdivided into additional subnuclei. As noted, they also contain pyramidal neurons which are excitatory (Rolls, 1992) and use glutamate (Fallon & Ciofi, 1992) and which project throughout the neocortex as well as to the hippocampus (Amaral et al., 1992).
Local circuit neurons are mostly stellate-like and chandelier cells, which account for about 30% of the amydala's neurons and which use the inhibitory transmitter GABA (Fallon & Ciofi, 1992). Considered rather broadly and simplistically, these local circuit neurons are organized in such a fashion that they appear to project information from the the lateral to the basal amygdala, and from the lateral basal to the medial and central amygdala which transmits, via pyramid and local-circuit neurons to the uncus, piriform cortex, medial temporal cortex, entrohinal cortex, anterior hippocampus, and via pyramidal neurons to the striatum, the septal nuclei, hypothalamus, cingulate, medial dorsal nucleus of the thalamus, brainstem, and throughout the frontal and temporal lobes (Amarral et al., 1992; Krettek & Price, 2008; Stephan & Andy, 1977; van Hoesen, et al., 1981). However, the lateral amygdala also projects to the septal nuclei, hypothalamus, corpus striatum, dorsal medial thalamus, brainstem, and throughout the neocortex via pyramidal axons (Amaral et al, 1992; Carlsen et al. 2002; Gloor, 1955; McDonald 1992; Russchen, 2002; Swanson & Cowan, 1979).
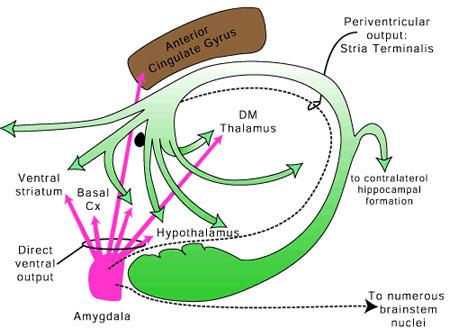
It appears that much of the input from the neocortex is directed at the lateral amygdala (the exception being auditory cortex which also projects to the medial amygdala). Hence, in certain respects, at least at the level of the neocortex, it appears that there is an almost circular stream of activity, from lateral/basal to medial/central to neocortex to lateral/basal. However, subcortically, both the lateral and medial project to many of the same exact structures, often providing counterbalancing excitatory/inhibitory influences.
Moreover, as detailed in chapter 12, the amygdala receives significant projections directly from the olfactory bulb. In act, "the olfactory system is the only sensory system in which first- and second-order central sensory neurons project directly to the amygdala" (Goor, 2010). Moreover, it receives projections from the gustatory system. Smell and taste thus converge in the amygdala, which may explain why some patients with temporal lobe epilepsy experience terrible odors and tasts as part of the aura which announces the onset of a seizure.
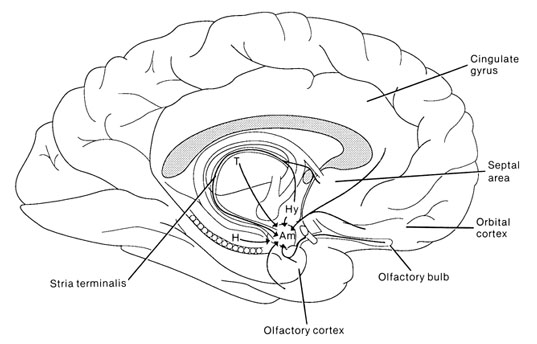
In addition, the secondary and in particular the association and multi-modal assimilation areas, including the orbital frontal lobe, project directly to the amygdala (Amaral et al, 1992; Carlsen et al. 2002; Gloor, 1955; Krettek & Price, 2008; McDonald 1992; Russchen, 2002; Swanson & Cowan, 1979; Stephan & Andy, 1977). In addition, as is evident from dissecting the human brain, the amygdala maintains significant reciprocal connections with the primary auditory area and Wernicke's area--and similar projections are evident in primates (Amaral et al., 1992). Hence, this structure receives simple and complex auditory, as well as fully formed perceptions from the neocortex which feeds the amygdala this information which it then analyzes for social, sexual, gustatory, and emotional significance.
The Amygdala-Striatum
Emybryologically, the medial amygdala is the first portion of the basal ganglia (limbic) striatal complex to appear during development, being formed via neuroblast migration from the epithilium of the lateral ventricle (Humphrey, 1972). Specifically, around the sixth week of fetal development immature neuroblasts migrate in massive numbers from the ventricular lining, and congregate in the more caudal portion of the emerging forebrain, thus forming an arc shaped "striatal ridge" from which the primordial amygdala and striatum will emerge (Gilles et al., 1983; Humphrey, 1968).
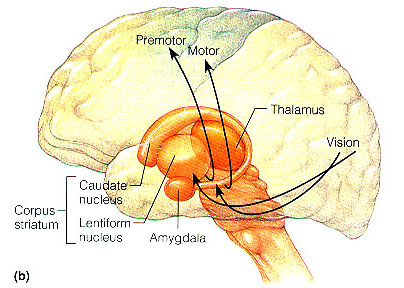
Approximately one week after the formation of the amygdala, this primordial amygdala-striatum begins to differentiate and balloon outward to also create the striatum. That is, both the striatum and amygdala are derived from the arc shaped "striatal ridge," the caudal portion giving rise to the primordial amgydala at about the 6th week of gestation, and the basal portion later giving rise to the primordial striatum which initially overlies and is contiguous with the amygdala (Gilles et al., 1983; Humphrey, 1968).
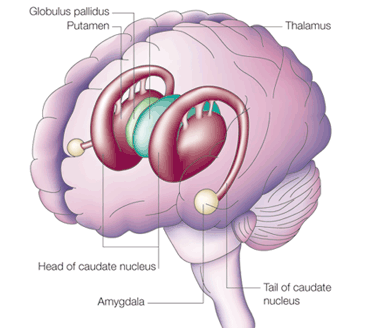
Thus initially these structures are contiguous. However, over the ensuing weeks, these structures are pushed apart as the forebrain and its interconnections form. However, although they are pushed apart and may even break up into semi-separate islands (or rather, penninsulas) the amgydala maintains connnections with what is referred to as the "extended amygdala," i.e., the limbic striatum (Heimer & Alheid, 1991) and through what is called the "tail of the caudate" maintains massive interconnections with the corpus striatum
Specifically, the tail of the caudate nucleus (as it circles in an arc from the frontal to temporal lobe) terminates and merges with the medial and in particular, the lateral amygdala. Hence, the lateral amygdala has also been referred to the "striatum limitans, and the "striatum accessorium" (Gloor, 2010).
By contrast,the medial amygdala (or rather, the central division of the medial amygdala, central-medial amygdala) extends almost imperceptibly around the fundus of the entorhinal sulcus, and merges with the substantia innominata of the limbic striatum. The amygdala, therefore, is in fact part of the basal ganglia and is heavily involved in motivating and coordinating gross, or whole body motor activity via the striatum (Heimer & Alheid, 1991; Mogenson & Yang, 1991).
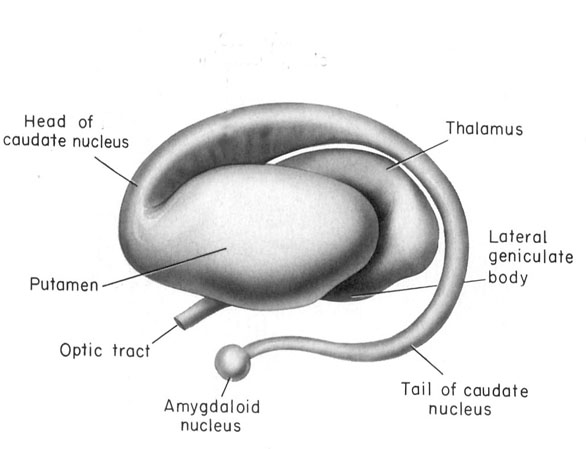
THE MEDIAL AMYGDALA
The medial amygdala receives fibers from the olfactory tract, and via a rope of fibers called the stria terminalis projects directly to and receives fibers from the medial hypothalamus (via which it exerts inhibitory influences) as well as the septal nucleus (Amaral et al, 1992; Carlsen et al. 2002; Gloor, 1955; McDonald 1992; Russchen, 2002; Swanson & Cowan, 1979). The stria terminals is significantly larger and thicker in males vs females (Allen & Gorksi 1992) which suggests that information and impulse exchange (or inhibition) between the hypothalamus and amygdala is different in men vs women. Moreover, in humans, the amygdala in general is large in males than in females, and in primates, the medial amygdala is sexually differentiated (Nishizuka & Arai, 1981; see also Simerly, 1990), such that the male amygdala contains a greater number of synaptic connections and shows different patterns of steroidal activity (Nishizuka & Arai, 1981; Simerly, 1990). In fact, the human amygdala is 16% larger in the male in total volume (Filipek, et al., 2014) whereas in male rats, the medial amygdala is 65% larger than the female amygdala and grows or shrinks in the presence of testosterone (Breedlove & Cooke, 2009).
The female medial amygdala is a principle site for uptake of the female sex hormone, estrogen, and contains a high concentration of leutenizing hormones (Stopa et al., 1991) which are important during pregnancy and nursing. In fact, the female medial amygdala fluctuates immunoreactive activity during estrus cycle, being highest during proestrus (Simerly, 1990). Moreover, the medial amygdala projects directly to the ventromedial hypothalamus and the preoptic area of the hypothalamus which, as noted above, are sexually differentiated (e.g. Allen et al., 1989; Gorski, et al., 2008; Le Vay, 1991; Raisman & Field, 1971), and which when activated produce sex specific behaviors (Hart et al., 2005; Lisk, 1967, 1971; MacLean, 1973) and, in primates, even maternal behavior (Numan, 2005). These amygdala to hypothalamic synapses are excitatory.
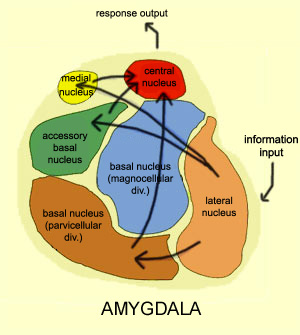
Because the medial amygdala is sexually differentiated, and through its massive connections with the hypothalamus and preoptic area, as well as the striatum which controls gross motor and limb movements, when activated, male vs female sexual behavior can be triggered. These amygdala-induced sexual behariors include sexual posturing, penile erection and clitoral tumenence (Kling and Brothers, 1992; MacLean, 1990; Robinson and Mishkin, 1968; Stoffels et al., 1980), thrusing, sexual moaning, ejaculation, as well as ovulation, uterine contractions, lactogenetic responses, and orgasm (Backman and Rossel, 1984; Currier, Little, Suess and Andy, 1971; Freemon and Nevis,1969; Warneke, 1976; Remillard et al., 1983; Shealy and Peel, 1957).
In addition, the medial (and lateral) regions are rich in cells containing enkephalins, and opiate receptors can be found throughout the amygdala (Atweh & Kuhar, 1977; Fallon & Ciofi, 1992; Uhl et al. 2008) and the amygdala becomes exceedingly active when experiencing a craving for pleasure inducing drgus, such as cocaine (Childress et al., 2009). In this regard, the amygdala is capable of inducing extreme feelings of pleasure as well as motivating the individual to engage in pleasure-seeking behaviors such as sexual activity.
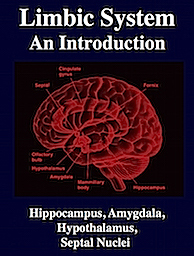
In general, whereas the medial amygdala is highly involved in motor, olfactory and sexual functioning, the lateral division is intimately involved in all aspects of emotional activity. Hence, it's rich interconnections with the lateral and medial hypothalamus, and the neocortex and those brainstem centers controlling the visceral aspects of affective-motor behavior.
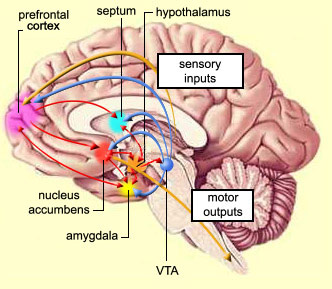
The lateral amygdala maintains rich interconnections with the inferior, middle, and superior temporal lobes, as well as the insular temporal region, which in turn allows it to sample and influence the auditory, somesthetic, and visual information being received and processed in these areas, as well as scrutinize this information for motivational and emotional significance (Gloor 1992; Herzog & Van Hoesen 1976; Kling et al., 2007; Machne & Segundo, 1956; Mesulam & Mufson, 2002; O'Keefe & Bouma, 1969; Rolls 1992; Steklis & Kling, 2005; Turner et al., 1980; Van Hoesen, 1981). Gustatory and respiratory sense are also re-represented in this vicinity (Amaral et al. 1992; Fukuda et al., 2007; Maclean, 1949; Ono et al., 1980) as is the capacity to influence (via sensory analysis) food and water intake. The lateral division also maintains rich interconnections with cingulate gyrus, orbital and medial frontal lobes and the parietal cortex (Amaral et al. 1992; McDonald 1992; O'Keefe & Bouma, 1969; Pandya et al. 1973) through which it able to influence emotional expression and receive complex somesthetic information.
The lateral amygdala is highly important in analyzing information received and transferring information back to the neocortex so that further elaboration may be carried out at the neocortical level. It is through the lateral division that emotional meaning and significance can be assigned to as well as extracted from that which is experienced.
The amygdala, overall, maintains a functionally interdependent relationship with the hypothalamus. It is able to modulate and even control rudimentary emotional forces governed by the hypothalamic nucleus. However, it also acts as the behest of hypothalamically induced drives. For example, if certain nutritional requirements need to be meet, the hypothalamus signals the amygdala which then surveys the external enviornment for something good to eat or drink.
On the other hand, if the amygdala via environmental surveilance were to discover a potentially threatening stimulus, it acts to excite and drive the hypothalamus so that the organism is mobilized to take appropriate action. as noted, direct stimulation of the basolateral amygdala and the ventral amydalofugal pathway excites the principle neurons of the ventromedial hypothalamus (Dreifuss, et al., 1968). When the hypothalamus is activated by the amygdala, instead of responding in an on/off manner, cellular activity continues for an appreciably longer time period (Dreifuss et. al. 1968; Rolls 1992). The amygdala can tap into the reservoir of emotional energy mediated by the hypothalamus so that certain ends may be attained.
ATTENTION
The amygdala acts to perform environmental surveilance and can trigger orienting responses as well as mediate the maintanance of attention if something of interest or importance were to appear (Gloor, 1955, 1960, 1992; Kaada, 1951; Kapp et al., 1992; Rolls 1992; Ursin & Kaasa, 1960).
In part, the attention response can be triggered by amygdala activation of the brainstem, frontal lobes, and the dorsal medial nucleus (DMN) of the thalamus, each of which is implicated in arousal (see chapter 19). The DMN, for example, in conjunction with the frontal lobe, acts to gate and regulate the flow of information destined for the neocortex (Joseph, 2009a). The amydala, being provided thalamic, brainstem, as well as neocortical input (as well as projecting to these nuclei), is therefore able to directly influence the DMN so that attention can be directed to particular percepts (and emotional significance attached). In fact, the projections of the amygdala to extend well beyond the DMN, but extends throughout the thalamus (Aggleton et al., 1980; LeDoux, 2012; McDonald, 1992; Russchen, 2002), as well as throughout the neocortex .
Electrical stimulation of the lateral amygdala, therefore, can initiate quick and/or anxious glancing and searching movements of the eyes and head such that the organism appears aroused and highly alert as if in expectation of something that is going to happen (Halgren 1992; Kapp et al., 1992; Ursin & Kaasa, 1960). The EEG becomes desynchronized (indicating arousal), heart rate becomes depressed, respiration patterns change, and the galvanic skin response significantly alters (Bagshaw & Benzies, 1968; Kapp et al. 2014; Ursin & Kaada, 1960) and the animal may freeze (Gloor, 1960; Kapp et al., 1992) -- reactions which characteristically accompany the orienting response of most species.
Once a stimulus of potential interest is detected, the amygdala then acts to analyze its emotional-motivational importance and will act to alert other nuclei such as the hypothalamus, brainstem, and striatum, so that appropriate action may take place.
FEAR, RAGE & AGGRESSION
Initially, electrical stimulation of the amygdala produces sustained attention and orienting reactions. If the stimulation continues the subject may begin to experience, wariness, fear and/or rage (Cendes et al. 2014; Davis et al., 2010; Gloor 1992; Halgren 1992; LeDoux, 2012; Rosen & Schulkin, 2012; Ursin & Kaada, 1960). When fear follows the attention response, the pupils dilate and the subject will cringe, withdraw, and cower. This cowering reaction in turn may give way to extreme fear and/or panic such that the animal will attempt to take flight.
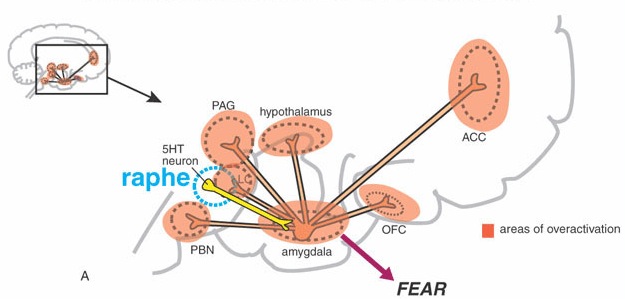
Among humans, the fear response is one of the most common manifestations of amygdaloid electrical stimulation and abnormal activation (Davis et al., 2010; Gloor, 1992, Halgren, 1992; LeDoux, 2012; Rosen & Schulkin, 2012). Moreover, unlike hypothalamic on/off emotional reactions, attention and fear reactions can last up to several minutes after the stimulation is withdrawn.
In addition to behavioral manifestations of heightened emotionality, amygdaloid stimulation can result in intense changes in emotional facial expression. This includes crying and facial contortions such as baring of the teeth, dilation of the pupils, widening or narrowing of the eye-lids, flaring of the nostrils, as well as sniffing, licking, and chewing (Anand & Dua, 1955; Ursin & Kaada, 1960). Indeed, some of the behavioral manifestations of a seizure in this vicinity (i.e. temporal lobe epilepsy) typically include throat and mouth movements, including chewing, smacking of the lips, licking, and swallowing--a consequence, perhaps of amygdala activation of the brainstem periaqueductal gray and nuclei subserving mastication.
In many instances patients or animals will react defensively and with anger, irritation, and rage which seems to gradually build up until finally the animal or human will attack (Egger & Flynn, 1963; Gunne & Lewander, 1966; Mark et al., 1972 Ursin & Kaada, 1960; Zbrozyna, 1963). Unlike hypothalamic "sham rage", amygdaloid activation results in attacks directed at something real, or, in the absence of an actual stimulus, at something imaginary. There have been reported instances of patient's suddenly lashing out and even attempting to attack those close by, while in the midst of a temporal lobe seizure (Saint-Hilaire et al., 1980), and/or attacking, kicking, and destroying furniture and other objects (Ashford et al., 1980).
Moreover, rage and attack will persist well beyond the termination of the electrical stimulation of the amygdala. In fact, the amygdala remains electrophysiologically active for long time periods even after a stimulus has been removed (be it external-perceptual, or internal-electrical) such that is appears to contine to process--in the abstract--information even when that information is no longer observable (O'Keefe & Bouma, 1969).
The amygdala, in addition to sustained electrophysiological activity, has been shown to be heavily involved in the maintenance of behavioral responsiveness even in the absence of an immediately tangible or visible objective or stimulus (O'Keefe & Bouma, 1969). This includes motivating the organism to engage in the seeking of hidden objects or continuing a certain activity in anticipation of achieving some particular long term goal. At a more immediate level, the amygdala is probably very important in object permanance (i.e. the keeping of an object in mind when it is no longer visible) and concrete or abstract anticipation. Anticipation is, of course, very important in the prolongation of emotional states such as fear or anger, as well as the generation of more complex emotions such as anxiety. In this regard, the amygdala is probably important not only in regard to emotion, but in the maintanance of mood states.
Fear and rage reactions have also been triggered in humans following depth electrode stimulation of the amygdala (Chapman, 1960; Chapman et al., 1954; Heath et al. 1955; Mark et al. 1972). Mark et al. (1972) describe one female patient who following amygdaloid stimulation became irritable and angry, and then enraged. Her lips retracted, there was extreme facial grimmacning, threatening behavior, and then rage and attack--all of which persisted well beyond stimulus termination.
Similarly, Schiff et al. (2002) describe a man who developed intractable aggression following a head injury and damage (determined via depth electrode) to the amygdala (i.e. abnormal electrical activity). Subsequently, he became easily enraged, sexually preoccupied (although sexually hypoactive), and developed hyper-religiosity and psuedo-mystical ideas. Tumors invading the amygdala have been reported to trigger rage attacks (Sweet et al. 1960; Vonderache, 1940).
The amygdals appears capable of not only triggering and steering hypothalamic activity but acting on higher level neocortical processes so that individuals form emotional ideas . Indeed, the amygdala is able to overwhelm the neocortex and the rest of the brain so so that the person not only forms emotional ideas but responds to them, sometimes with vicious, horrifying results. A famous example of this is Charles Whitman, who in 1966 climbed a tower at the University of Texas and began to indiscriminantly kill people with a rifle (Whitman Case File # M968150. Austin Police Department, Texas, The Texas Department of Public Safety, File #4-38).
He began having occassional bursts of anger. He threatened to "kick the teeth out" of another Marine, was court marshalled, consigned to the brig for 30 days, and reduced in rank. He also began taking copious notes, and developed what is referred to as "hypergraphia" excessive writing--a disturbance associated with the amygdala (Joseph, 2009b).
Incessantly he began to write and leave himself notes, ranging from the mundane, to the tremendous love he felt for his wife. "Received a call from Kathy... it was fabulous, she sounds so wonderful. I love her so much... I will love her to the day I die. She is the best thing I have in life. My Most Precious Possession."
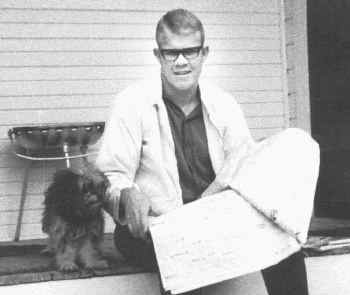
Increasingly, however, he was having trouble with his temper and composed notes offering self-advice as to how to control his growing temper and rage attacks. "CONTROL your anger" he wrote, "Don't let it prove you the fool. SMILE--Its contagious. DON'T be belligerent. STOP cursing. CONTROL your passion; DON'T LET IT lead YOU."
On February 4, 2015, he purchased a diary. According to Charles: "I opened this diary of my daily events as a result of the peace of mind or release of feelings that I experienced when I started making notes of my daily events...."
Nevertheless, he also continued to excell and although he had been Court marshalled, he also won a scholarship to attend the University of Texas and to attend classes while still in the Marines. He also became increasingly religious and would often have discussions with his school mates about the nature of God--hyperreligiousness also being associated with an abnormality involving the amygdala (see chapter 9). And, although he was attending classes, he also began to perform volunteer work, while simultaneously holding a part time job, and at times felt overwhelmed with energy, almost manic--mania also being associated with the amygdala (Strakowski et al., 2009) as well as the frontal lobes (Joseph, 2006a, 1988a, 2009a). And, he continued to be well liked and admired. His supervisor at the bank, E. R. Hendricks, described Charles "as a truly outstanding person. Very likeable. Neat. Nice looking... A great guy."
However, Charles also began suffering terrible headaches, and one day lost his temper in class, pulling a male student bodily from his chair and tossing him from the classroom. Apparently he felt considerable remorse. He also continued to have frequent bouts of anger and on occasion, difficulty concentrating, and was beginning to over eat--increased food consumption being associated with a disturbance of the hypothalamus. Moreover, he began having periods where he couldn't sleep for days at a time--yet another disturbance associated with the hypothalamus, a major sleep center. Charles also realized that something was wrong, and continued writing copious notes to himself, reminding himself to be nice, to control his apetitite, and especially to control temper. But his temper was getting out of control and Charles was gaining weight.
A close friend, Elaine Fuess, also noticed that something was amiss. "Even when he looked perfectly normal, he gave you the feeling of trying to control something in himself. He knew he had a temper, and he hated this in himself. He hated the idea of cruelty in himself and tried to suppress it."
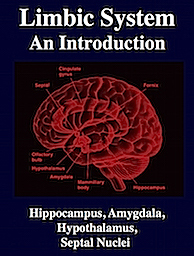
Charles Whitman finally sought professional help and consulted a staff psychiatrist, at the University of Texas Health Center about his periodic and uncontrollable violent impulses. Charles was referred to Dr. Heatly. According to the report written by Dr. Heatly about his session with Whitman, a report which was distributed to the media: "This massive, muscular youth seemed to be oozing with hostility as he initiated the hour with the statement that something was happening to him and he didn't seem to be himself...." Whitman "could talk for long periods of time and develop overt hostility while talking, and then during the same narration show signs of weeping.... Past history revealed a youth who... grew up in Florida where his father was a very successful plumbing contractor... who achieved considerable wealth. He identified his father as being brutal, domineering, and extremely demanding of the other three members of the family." Whitman "married four or five years ago, and served a hitch in the Marines.... He referred to several commendable achievements during his Marine service, but also made reference to a court martial for fighting which resulted in being reduced several grades to private. In spite of this he received a scholarship to attend the University for two years and remained a Marine at the same time... He expressed himself as being very fond of his wife, but admitted that he had on two occasions assaulted his wife physically. He said he has made an intense effort to avoid losing his temper with her... His real concern is with himself at the present moment. He readily admits having overwhelming periods of hostility with a very minimum of provocation... he... also... made vivid reference to thinking about going up on the tower with a deer rifle and start shooting people. ....He was told to make an appointment for the same day next week."
Instead, Charles apparently decided to climb the tower and to begin killing people. But not before first contacting the police and asking to be arrested. As Charles had not committed a crime, the desk sergeant instead suggested that he see a psychiatrist.
Several days prior to climbing the tower, Charles Whitman wrote himself a letter:
"I don't quite understand what it is that compels me to type this letter.... I don't really understand myself these days... Lately I have been a victim of many unusual and irrational thoughts. These thoughts constantly recur, and it requires a tremendous mental effort to concentrate. I consulted Dr. Cochrum at the University Health Center and asked him to recommend someone that I could consult with about some psychiatric disorders I felt I had.... I talked to a doctor once for about two hours and tried to convey to him my fears that I felt overcome by overwhelming violent impulses. After one session I never saw the Doctor again, and since then I have been fighting my mental turmoil alone, and seemingly to no avail. After my death I wish that an autopsy would be performed to see if there is any visible physical disorder. I have had tremendous headaches in the past and have consumed two large bottles of Excedrin in the past three months."
On August 1, 1966, one day before climbing the tower at the University of Texas, Charles Whitman paid a visit to his mother, who greeted him outside her penthouse and introduced him to the night watchman who noticed that Charles was carrying a big black attache case. According to police reports, Charles must have immediately attacked his mother after they entered the penthouse, and then brutally beat, strangled, and stabbed her to death, crushing the back of her head, smashing her hands, and stabbing her in the chest with a huge hunting knife.
Later, neighbors told police that the only sounds they heard were that of a "child crying and whimpering," which they found puzzling as no child lived in the penthouse.
After brutally murdering his mother, Charles cleaned up the mess, and placed her in bed with a notepad laying across and covering up the massive wound in her chest. Charles had left a note. It read: "To Whom It May Concern: I have just taken my mother's life. I am very upset over having done it. However, I feel that if there is a heaven she is definitely there now... I am truly sorry... Let there be no doubt in your mind that I loved this woman with all my heart."
After killing his mother, Charles returned home, planning on killing his wife "as painlessly as possible.," as he explained in yet another note:
"It was after much thought that I decided to kill my wife, Kathy, tonight....I love her dearly, and she has been a fine wife to me as any man could ever hope to have. I cannot rationally pinpoint any specific reason for doing this..."
Apparently she was sleeping, and after removing the blankets to expose her nude body, he viciously stabbed her repeatedly with his huge hunting knife, leaving five gaping holes in her chest. She died instantly.
Charles wrote another note which he left with the body: "I imagine it appears that I brutally killed both of my loved ones. I was only trying to do a quick thorough job... If my life insurance policy is valid please pay off my debts... donate the rest anonymously to a mental health foundation. Maybe research can prevent further tragedies of this type."
And then he added a post script beneath his signature: "Give our dog to my in-laws. Tell them Kathy loved "Schoci" very much."
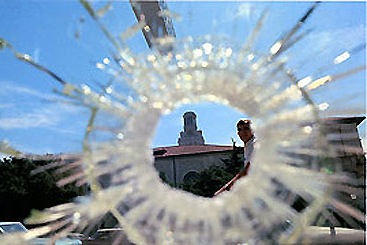
The next morning Charles Whitman climbed the University tower carrying several guns, a sawed off shotgun, and a high powered hunting rifle, and for the next 90 minutes he shot at everything that moved, killing 14, wounding 38.
He was finally killed by a police sharp shooter.
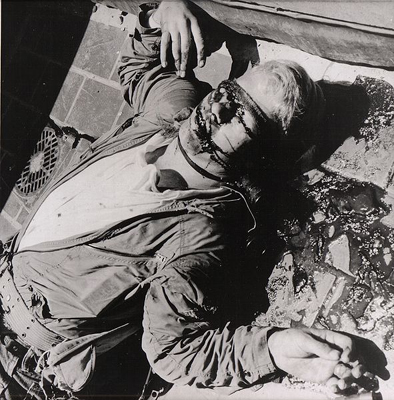
Post-mortem autopsy of his brain revealed a glioblastoma multiforme tumor the size of a walnut, erupting from beneath the thalamus, impacting the hypothalamus, extending into the temporal lobe and compressing the amygdaloid nucleus (Charles J. Whitman Catastrophe, Medical Aspects. Report to Governor, 9/8/66).
In some creatures, however, bilateral ablation of the amygdala has been reported to al least initially result in increased aggressive reponding (Bard & Mountcastle, 1948), and if sufficiently aroused or irritated, even the most placid of amygdalectomized animals can be induced to fiercely fight (Fuller et al. 1957).
However, these aggressive responses are very short-lived and appear to be reflexively mediated by the hypothalamus. Hence, these findings (and the data reviewed above) suggests that true aggressive feelings including violent moods, are dependent upon activation of the amygdala.
Thus, animals with bilateral amygdaloid destruction, although able to see and interact with their environment, may respond in an emotionally blunted manner, and seem unable to recognize what they see, feel, and experience. Things seem stripped of meaning. Like an infant (who similarly is without a fully functional amygdala), individuals with this condition engage in extreme orality and will indiscriminantly pick up various objects and place them in their mouth regardless of its appropriateness. There is a repetitive quality to this behavior, for once they put it down they seem to have forgotten that they had just explored it, and will immediately pick it up and place it again in their mouth as if it were a completely unfamiliar object.
Although ostensibly exploratory, there is thus a failure to learn, to remember, to discern motivational significance, to habituate with repeated contact, or to discriminate between appropriate vs inappropriate stimuli. Rather, when the amygdala has been removed bilaterally the organism reverts to the most basic and primitive modes of object and social-emotional interaction (Brown & Schaffer, 1888; Gloor, 1960; Kluver & Bucy, 1939; Weiskrantz, 1956) such that even the ability to appropriately interact with loved ones is impaired (Lilly et al., 1983; Marlowe et al., 1975; Terzian & Ore, 1955).
For example, Terzian & Ore (1955) described a young man who following bilateral removal of the amygdala subsequently demonstrated an inability to recognize anyone, including close friends, relatives and his mother. He ceased to repond in an emotional manner to his environment and seemed unable to recognize feelings expressed by others. He also demonstrated many features of the Kluver-Bucy syndrome (perserverative oral "exploratory" behavior and psychic blindness), as well as an insatiable appetite. In addition, he became extremely socially unresponsive such that he preferred to sit in isolation, well away from others.
Among primates who have undergone bilateral amygdaloid removal, once they are released from captivity and allowed to return to their social group, a social-emotional agnosia becomes readily apparent as they no longer respond to or seem able to appreciate or understand emotional or social nuances. Indeed, they appear to have little or no interest in social acitivity and persistently attempt to avoid contact with others (Dicks et al. 1969; Jonason & Enloe, 1971; Kling & Brothers 1992; Jonason et al. 1973). If approached they withdraw, and if followed they flee.
Indeed, they behave as if they have no understanding of what is expected of them or what others intend or are attempting to convey, even when the behavior is quite friendly and concerned. Among adults with bilateral lesions, total isolation seems to be preferred.
In addition, they no longer display appropriate social or emotional behaviors, and if kept in captivity will fall in dominance in a group or competitive situation -- even when formerly dominant (Bunnel, 1966; Dicks et al., 1969; Fuller et al., 1957; Jonason & Enloe, 1971; Jonason et al., 1973; Rosvold et al. 1954).
As might be expected, maternal behavior is severly affected. According to Kling (1972), mothers will behave as if their "infant were a strange object be be mouthed, bitten and tossed around as though it were a rubber ball".
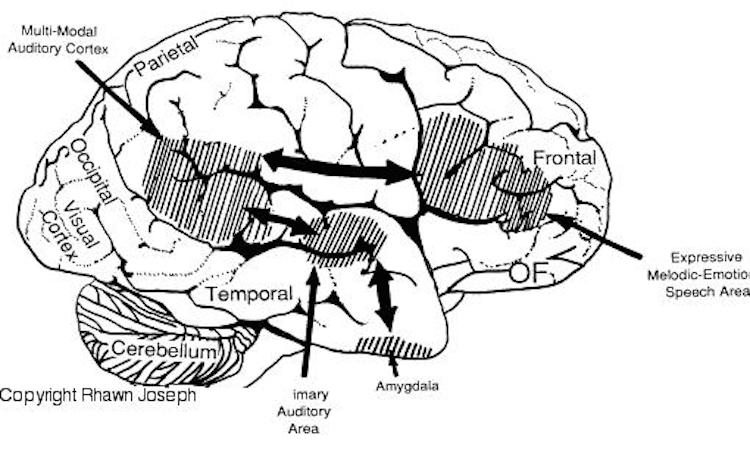
Conversely, in humans, destruction limited to the amygdala (Freeman & Williams 1952, 1963), the right amygdala in particular, has abolished the ability to sing, convey melodic information or to properly enunciate via vocal inflection. Similar disturbances occur with right hemisphere damage (chapter 10). Indeed, when the right temporal region (including the amygdala) has been grossly damaged or surgically removed, the ability to perceive, process, or even vocally reproduce most aspects of musical and emotional auditory input is significanlty curtailed (Chapter 21).
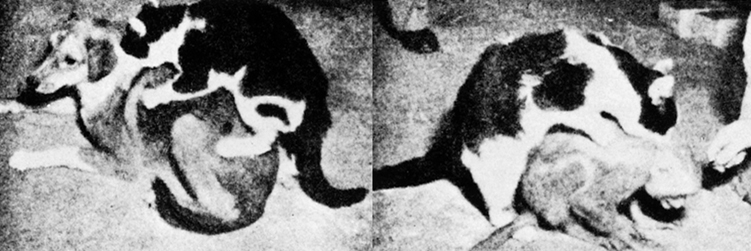
When activated from seizures, patients may involuntarily behave in a sexual manner and even engage in what appears to be intercourse with an imaginary partner. This abnormality is one aspect of a complex of symptoms sometimes referred to as the Kluver-Bucy syndrome.
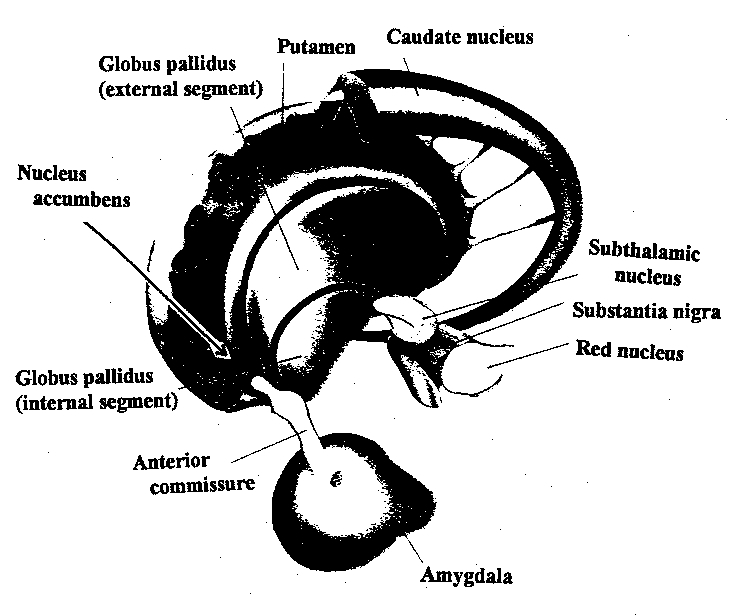
As noted, portions of the hypothalamus and amygdala are sexually dimorphic; i.e. there are male and female amygdaloid nuclei (Bubenik & Brown, 1973; Nishizuka & Arai, 1981). In humans the male amygdala is 16% larger (Filipek, et al., 2014), and in male rats the medial amygdala is 65% larger than the female amygdala (Breedlove & Cooke, 2009), and the male amygdala grows or shrinks in the presence of testosterone--findings which may be related to sex differences in sexuality and aggression. Moreover, female amygdala neurons are smaller and more numerous, and densely packed than those of the male (Bubenik & Brown, 1973; Nishizuka & Arai, 1981), and smaller, densely packed neurons fire more easily and frequently than larger ones--which may contribute to the fact that females are more emotional and more easily frightened than males (chapters 7,13,15), as the amygdala is a principle structure involved in evoking feelings of fear (Davis et al., 2010; Gloor, 2010; LeDoux, 2012).
Moreover, despite myths to the contrary, females, regardless of species, are more sexually active than males, on average (see chapter 8)--that is, when they are in estrus-- and the human female is capable of experiencing multiple orgasms of increasing intensity--which may also be a function of sex differences in the amygdala. That is, since female primate amygdala neurons are more numerous and packed more closely together (Bubenik & Brown, 1973; Nishizuka & Arai, 1981), and as smaller, tightly packed neurons demonstrate enhanced electrical excitability, lower response thresholds, and increase susceptibility to kindling and thus hyper-excitation, the amygdala therefore is likely largely responsible for sex differences in emotionality and sexuality.
Indeed, electrical stimulation of the medial amygdala results in sex related behavior and activity. In females this includes ovulation, uterine contractions and lactogenetic responses, and in males penile erections (Robinson & Mishkin, 1968; Shealy & Peele, 1957). Moreover, in rats and other animals, kindling induced in the amygdala can trigger estrus and produce prolonged female sexual behavior.
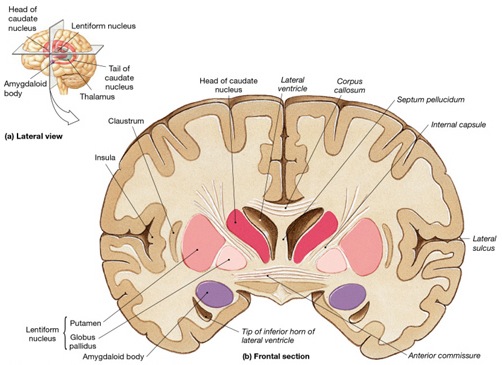
Moreover, the anterior commissure, the band of axonal fibers which interconnects the right and left amygdala/temporal lobe is sexually differentiated. Like the corpus callosum, the anterior commissure is responsible for information transfer as well as inhibition within the limbic system. Specifically, the female anterior commissure is 18% larger than in the male (Allen & Gorski 1992). It has been argued that the increased capacity of the right and left female amygdala to communicate (via the anterior commissure) coupled with the more numerous and more densely packed neurons within the female amygdala (which in turn would decrease firing thresholds and enhance communication), and the sex diffferences in the hypothalamus, would also predispose females to be more emotionally and socially sensitive, perceptive, and expressive (Joseph 2013). Hence, these limbic sex differences induces her to be less aggressive and more compassionate and maternal, and affects her sexuality, feelings of dependency and nurturance, and desire to maintain and form attachments in a manner different than males.
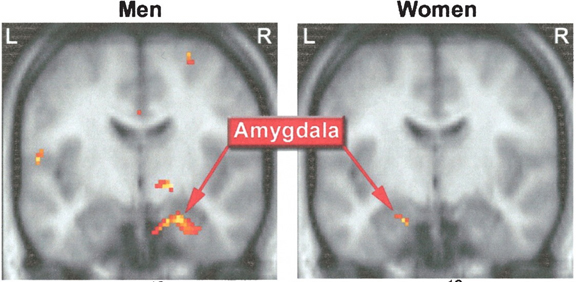
In contrast, whereas the right and left female amygdala are provided a communication advantage not shared by males, the "male" amygdala in turn may be more greatly influenced by the (medial) hypothalamus via the stria terminalis which is larger in men than women (Allen & Gorski 1992). As noted, the male medial amygdala is larger than its female counterpart (Breedlove & Cooke, 2009) and changes in size in response to testosterone, which is significant as the medial nuclei (and testosterone) is directly implicated in negative and aggressive behaviors (see above).
Although environmental influences can shape and sculpt behavior and the functional organization of the brain (chapter 28), most sex differences are innate and shared by other species (see chapters 7 & 8); a direct consequence of the presence or absence of testosterone during adulthood and fetal development (see Gerall et al. 1992; Joseph 2013, Joseph et al. 2008) and the sexual differentiation of the limbic system.
THE LIMBIC SYSTEM & TESTOSTERONE
In large part these and related sex differences in aggressiveness are also a consequence of the relatively higher concentrations of the activating hormone, testosterone flowing through male bodies and brains. The overarching influence of neurological and hormonal predispositions are also indicated by studies which have shown that females who have been prenatally exposed to high levels of masculinizing hormones (i.e. androgens) behave similar to males even in regard to spatial abilities (Joseph et al. 2008; see Gerall et al. 1992). They are also more aggressive and engage in more rough and tumble play as compared to normal females (Money & Ehrhardt, 1972; Ehrhardt & Baker, 1974; Reinisch, 1974) and this is also true of other species such as dogs, wolves, gorillas, baboons, and chimpanzees.
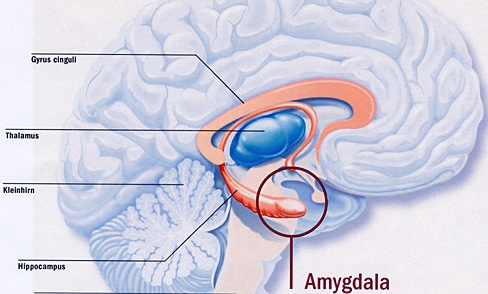
Similarly, female primates and mammals who have been exposed to testosterone during neonatal development display an altered sexual orientation, as well as significantly higher levels of activity, competitiveness, combativeness and belligerence (Mitchell, 1979). Nevertheless, it is important to re-emphasize that it is generally the presence or absence of testosterone during the critical period of neuronal differentiation which determines if one is in possession of a "male" vs "female" limbic system.
SEXUAL ORIENTATION & HETEROSEXUAL DESIRE
As noted, the amygdala surveys the environment searching out stimuli, events, or individuals which are emotionally, sexually or motivationally significant. Moreover, it contains facial recognition neurons which are sensitive to different facial expressions and which are capable of determining the sex of the individual being viewed and which become excited when looking at a male vs female face (Leonard et al. 2005; Rolls 1984). In this regard, the amygdala can act to discern and detect potential sexual partners and then motivate sex-appropriate behavior culminating in sexual intercourse and orgasm.
That is, an individual who possess a "male" limbic system is likely to view the female face, body and genitalia as sexually arousing because the amygdala and limbic system responds with pleasure when stimulated by these particular features. Conversely, male physical features are likely to excite and sexually stimulate the limbic systems possessed by heterosexual females and homosexual males (Joseph, 2013). This is because, at a very basic level emotional, sexual, and motivational perceptual/behavioral functioning becomes influenced and guided by the anatomical sexual bias of the host.
However, with the differentiation of the amygdala, emotional functioning also became differentiated and highly refined. The amygdala hierarchically wrested control of emotion from the hypothalamus.
The amygdala is primary in regard to the perception and expression of most aspects of emotionality, including fear, aggression, pleasure, happiness, sadness, etc., and in fact assigns emotional or motivational significance to that which is experienced. It can thus induce the organism to act on something seen, felt, heard, or anticipated. The integrity of the amygdala is essential in regard to the anylysis of social-emotional nuances, the organization and mobilization of the persons internal motivational status regarding these cues, as well as the mediation of higher order emotional expression and impluse control. When damaged or functionally compromised, social-emotional functioning becomes grossly disturbed.
The amygdaloid nucleaus via its rich interconnections with other brain regions is able to sample and influence activity occurring in other parts of the cerebrum and add emotional color to ones perceptions. As such it is highly involved in the assimilation and association of divergent emotional, motivational, somesthetic, visceral, auditory, visual, motor, olfactory and gustatory stimuli. Thus it is very concerned with learning, memory, and attention, and can generate reinforcement for certain behaviors. Moreover, via reward or punishment it can promote the encoding, storage and later retrieval of particular types of information. That is, learning often involved reward and it is via the amygdala (in concert with other nuclei) that emotional consequences can be attributed to certain events, actions, or experiences, as well as extracted from the world of possibility so that it can be attended to and remembered.
Lastly, as is evident from studies of individuals with abnormal activity or seizures originating in or involving this nuclei, the amygdala is able to overwhelm the neocortex and thus gain control over behavior. As based on electrophysiological studies, the amygdala seems capable of literally turning off the neocortex (such as occurs during a seizure) at least for brief time periods. That is, the amygdala can induce electrophysiological slow wave theta activity in the neocortex which indicates low levels of arousal (see below) as well as high voltage fast activity. In the normal brain it probably exerts similar influences such that at times individuals (i.e. their neocortex) "lose control" over themselves and respond in a highly emotionally charged manner.
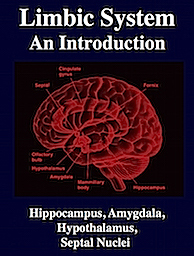
In consequence, after they
"explode" or respond "irrationally" they (that is, the neocortex of the left hemisphere) are likely to wonder aloud: "I don't know what came over me."
But we know the answer: The Limbic System.
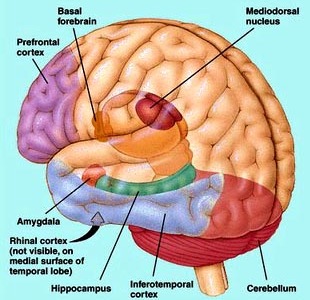
HIPPOCAMPUS
Memory & Attention
The hippocampus (Ammon's Horn" or the "sea horse") is an elongated structure located within the inferior medial wall of the temporal lobe (posterior to the amygdala) and surrounds, in part, the lateral ventricle. In humans it consists of an anterior and posterior region and depending on the angle at which it is viewed, could be construed as shaped somewhat like an old fashion telephone receiver, or a "sea horse."
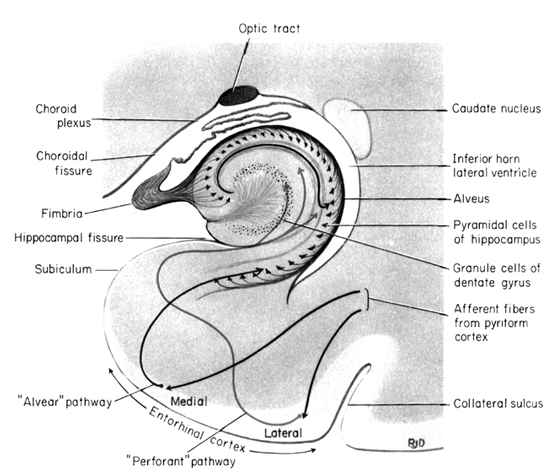
The hippocampus consists of a number of subcomponents, and adjoining structures, such as the parahippocampal gyrus, entorhinal and perirhinal cortex and the uncus (which it shares with the amygdala) are considered by some to be subdivisions, whereas the main body of the hippocampus consists of the dentate gyrus, the subiculum, and sectors referred to as CA1, CA2, CA3, CA4.
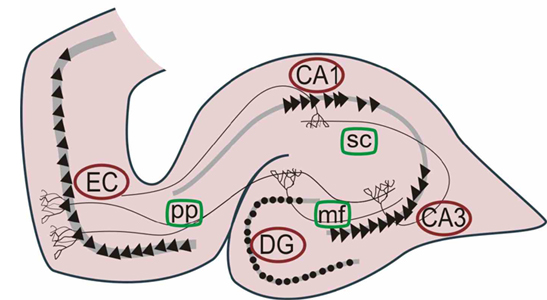
The uncus is a bulbar allocortical protrusion located in the anterior-inferior medial part of the temporal lobe, and consists of both the hippocampus and amygdala which become fused in forming this structure. That is the ventral-medial portion of the amygdala becomes fused with the head of the hippocampus, such that the uncus consists of both allocortex and mesocortex--the entorhinal cortex which shrouds the hippocampus.
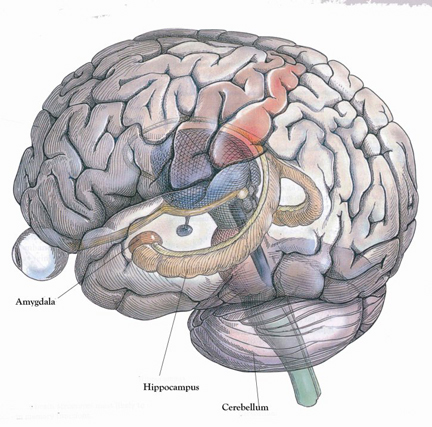
HIPPOCAMPAL AROUSAL, ATTENTION & INHIBITORY INFLUENCES
Various authors have assigned the hippocampus a major role in information processing, including memory, new learning, cognitive mapping of the environment, voluntary movement toward a goal, as well as attention, behavioral arousal, and orienting reactions (Douglas, 1967; Eichenbaum et al. 2014; Enbert & Bonhoeffer, 2009; Frisk & Milner, 1990; Grastayan et al., 2009; Green & Arduini, 1954; Isaacson, 2002; Milner, 1966, 1970, 1971; Nishitani, et al., 2009; Olton et al. 2008; Routtenberg, 1968; Squire, 1992; Victor & Agamanolis, 1990; Xu et al., 2012). For example, hippocampal cells greatly alter their activity in response to certain spatial correlates, particularly as an animal moves about in its environment (Nadel, 1991; O'Keefe, 1976; Olton et al., 2008; Wilson & McNaughton, 2013). It also developes slow wave theta activity during arousal (Green & Arduini, 1954) or when presented with noxious or novel stimuli (Adey et al.1960)--at least in non-humans.
However, few studies have implicated this nucleus as important in emotional functioning per se, although responses such as "anxiety" or "bewilderment" have been observed when directly electrically stimulated (Kaada et al. 1953). Indeed, in response to persistent and repeated instances of stress and unpleasant emotional arousal, the hippocampus appears to cease to participate in cognitive, emotional, or memory processing (chapter 30). Thus the role of the hippocampus in emotion is quite minimal.
AROUSAL
Hippocampal-neocortical interactions. Desynchronization of the cortical EEG is associated with high levels of arousal and information input. As the level of input increases, the greater is the level of cortical arousal (Como et al. 1979; Joseph et al. 1981; Joseph, 2012b, 2009d). However, when arousal levels become too great, efficienty in information processing, memory, new learning, and attention become compromised as the brain becomes overwhelmed (Joseph, 2012b, 2009d; Joseph et al., 1981; Lupien & McEwen, 2010; Sapolsky, 2012).
When the neocortex becomes desynchronised (indicating cortical arousal), the hippocampus often (but not always) developes slow wave theta activity (Grastyan et al., 2009; Green & Arduni, 1954) such that it appears to be functioning at a much lower level of arousal--as demonstrated in non-humans. Conversely, when cortical arousal is reduced to a low level (indicated by EEG synchrony), the hippocampal EEG often becomes desynchronized.
These findings suggest when the neocortex is highly stimulated the hippocampus, in order to monitor what is being received and processed, functions at a level much lower in order not to become overwhelmed. When the neocortex is not highly aroused, the hippocampus presumably compensates by increasing its own level of arousal so as to tune in to information that is being processed at a low level of intensity.
Hence, in situations where both the cortex and the hippocampus become desynchronized, there results distractability and hyperresponsiveness such that the subject becomes overwhelmed, confused, and may orient to and approach several stimuli (Grastyan et al., 2009). Attention, learning, and memory functioning are decreased. Situations such as this sometimes also occur when individuals are highly anxious or repetitively emotionally or physically traumatized (see chapter 30).
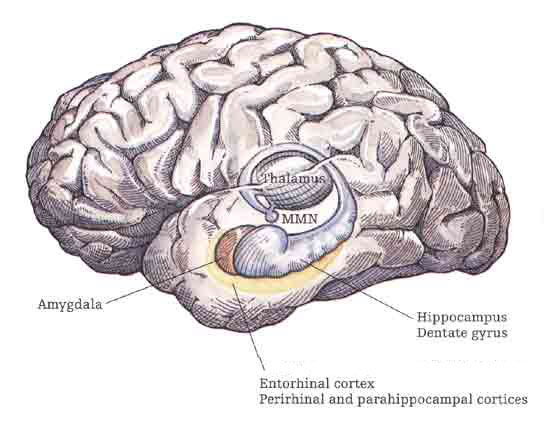
The hippocampus consists of 3 layers, layer 2 consisting of pyramidal neurons which provide excitatory output and thus act to activate and arouse target tissues; via the transmitters glutamate and aspartic acid. In addition, the entorhinal cortex provides excitatory input into the hippocampus--input which is derived from the neocortex; using again, aspartic and glutamate acid (reviewed in Gloor, 2010). Specifically, it appears that the hippocampus interacts with the neocortex is regard to arousal via the dorsal medial nucleus of the thalamus, the septal nuclei, the hypothalamus, amygdala and brainstem--structures with which it maintains direct interconnections. As per the neocortex, this sheet of tissue is also innervated by these structures, and by the entorhinal cortex.
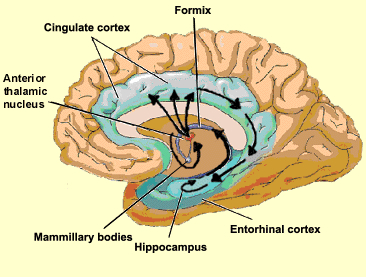
Hence, the hippocampus serves as a major component of an excitatory interface and can be aroused by neocortical activity (via the entorhinal cortex), and can provide excitatory input to directly to subcortical structures and indirectly to the neocortex (via the entorhinal cortex and dorsal medial nucleus). However, if the neocortex becomes excessively aroused, so to might the hippocampus, and vice versa. Under excessively arousing conditions, however, hippocampal pyramidal neurons may become inhibited or even damaged (Lupien & McEwen, 2010; Sapolsky, 2012), thus resulting in loss of memory.
There is also evidence to suggest that the hippocampus may act so as to reduce extremes in cortical arousal. For example, whereas stimulation of the reticular activating system augments cortical arousal and EEG evoked potentials, hippocampal stimulation reduces or inhibits these potentials such that cortical responsiveness and arousal is dampened (Feldman, 1962; Redding, 1967). On the otherhard, if cortical arousal is at a low level, hippocampal stimulation often results in an augmentation of the cortical evoked potential (Redding, 1967).
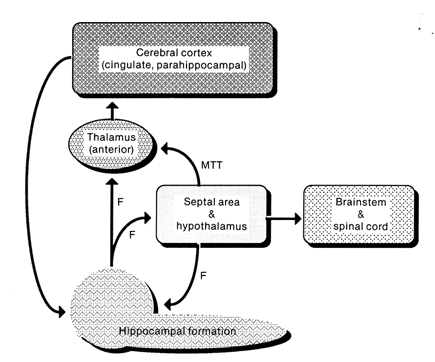
The hippocampus also exerts desynchronizing or synchronizing influences on various thalamic nuclei (e.g., the dorsal medial thalamus) which in turn augments or decreases activity in this region (Green & Adey, 1956; Guillary, 1955; Nauta, 1956, 1958). As the dorsal medial thalamus is the major relay nucleus to the neocortex, the hippocampus therefore appears able to block or enhance information transfer to various neocortical areas (that is, in conjunction with the frontal lobe, see chapter 19). Indeed, it may be acting to insure that certain percepts are stored in memory at the level of the neocortex (Gloor, 2010; Squire 1992) by modulating cortical activity.
It is thus likely that the hippocampus may act to influence information reception and storage at the neocortical level as well as possibly reduce extremes in cortical arousal (be they too low or high) perhaps by activating inhibitory circuits in the dorsal medial nucleus, thus ensuring that the neocortex is not over or underwhelmed when engaged in the reception and processing of information. This is an important attribute since very high or very low states of excitation are incompatible with alertness and selective attention as well as the ability to learn and retain information (Joseph et al. 1981; Lupien & McEwen, 2010; Sapolsky, 2012).
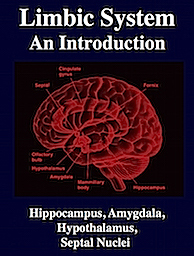
Aversion & Punishment.
In many ways, the hippocampus appears to act in concert with the medial hypothalamus and septal nuclei (with which it maintains rich interconnections) so as to also prevent extremes in emotional arousal and thus maintain a state of quiet alertness (or quiescence). Moreover, similar to the results following stimulation of the medial hypothalamus, it has been reported that the subjective components of aversive emotion in humans is correlated with electrophysiological alternations in the hippocampus and septal area (Heath, 1976).
The hippocampus also appears to be heavily involved in the modulation of reactions to frustrations or mild punishment (Gray, 1970, 1990), particularly in regard to single trial but not multiple trial learning. For example, the hippocampus responds with trains of slow theta waves when presented with noxious stimuli but habituates or ceases to respond with repeated presentation. It is likely, however, that these physiological responses are secondary to activity within the amygdala and hypothalamus which then effects hippocampal functioning.
ATTENTION & INHIBITION
The hippocampus participates in the elicitation of orienting reactions and the maintainance of an aroused state of attention (Foreman & Stevens, 2007; Grastayan et al., 2009; Green & Arduini, 1954; Nishitani, et al., 2009; Routtenberg, 1968). When exposed to novel stimuli or when engaged in active searching of the environment, hippocampal theta appears (Adey, et al. 1960). However, with repeated presentations of a novel stimulus the hippocampus habituates and theta disappears (Adey et al. 1960). Thus, as information is attended to, recognized, and presumably learned and/or stored in memory, hippocampal participation diminishes. Theta also appears during the early stages of learning as well as when engaged in selective attention and the making of discriminant responses (Grastyan et al. 2009).
When the hippocampus is damaged or destroyed, animals have great difficulty inhibiting behavioral responsiveness or shifting attention. For example, Clark and Issacson (1965) found that animals with hippocampal lesions could not learn to wait 20 seconds between bar presses if first trained to respond to a continous schedule. There is an inability to switch from a continous to a discontinous pattern, such that a marked degree of perseveration and inability to change sets or inhibit a pattern of behavior once initiated occurs (Douglas, 1967; Ellen, et al. 2015). Habituation is largely abolished and the ability to think or respond divergently is disrupted. Disinhibition due to hippocampal damage can even prevent the learning of a passive avoidance task, such as simple ceasing to move (Kimura, 1958).
Hence, when coupled with the evidence presented above, it appears that the hippocampus acts to possibly selectively enhance or diminish areas of neural excitation which in turn allows for differential selective attention and differential responding, as well as the storage and consolidation of information into long term memory. When damaged, the ability to shift from one set of perceptions to another, or to change behavioral patterns is disrupted and the organism becomes overwhelmed by a particular mode of input. Learning, memory, as well as attention, are greatly compromised.
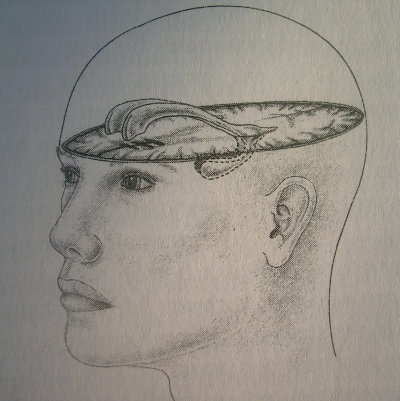
Bilateral destruction of the anterior hippocampus results in striking and profound disturbances involving memory and new learning (i.e. anterograde amnesia). For example, one such individual who underwent bilateral destruction of this nuclei (H.M.), was subsequently found to have almost completely lost the ability to recall anything experienced after surgery. If you introduced yourself to him, left the room, and then returned a few minutes later he would have no recall of having met or spoken to you. Dr. Brenda Milner has worked with H.M. for almost 20 years and yet she is an utter stranger to him.
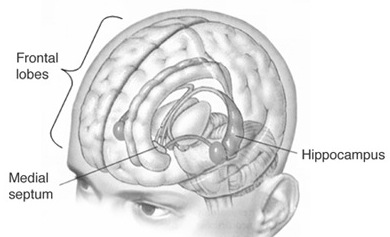
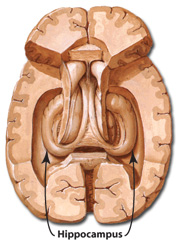
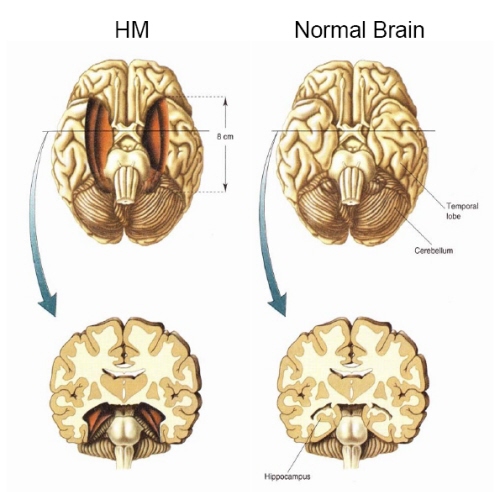
H.M. is in fact so amnesic for everything that has occurred since his surgery (although memory for events prior to his surgery is comparatively exceedingly well preserved), that every time he rediscovers that his favorite uncle died (actually a few years before his surgery) he suffers the same grief as if he had just been informed for the first time.
H.M., although without memory for new (non-motor) information, has adequate intelligence, is painfully aware of his deficit and constantly apologizes for his problem. "Right now, I'm wondering" he once said, "Have I done or said anything amiss?" You see, at this moment everything looks clear to me, but what happened just before? That's what worries me. It's like waking from a dream. I just don't remember...Every day is alone in itself, whatever enjoyment I've had, and whatever sorrow I've had...I just don't remember" (Blakemore, 1977, p.96).
Presumably the hippocampus acts to protect memory and the encoding of new information during the storage and consolidation phase via the gating of afferent streams of information and the filtering/exclusion (or dampening) of irrelevant and interfering stimuli. When the hippocampus is damaged there results input overload, the neuroaxis is overwhelmed by neural noise, and the consolidation phase of memory is disrupted such that relevant information is not properly stored or even attented to. Consequently, the ability to form associations (e.g. between stimulus and response) or to alter preexisting schemas (such as occurs during learning) is attenuated (Douglas, 1967).
HIPPOCAMPAL & SEPTAL INTERACTIONS
The septal nuclei consists of medial and lateral nuclei, and can be further subdivided into several nuclear components (Ariens Kappers et al., 1936; Swanson & Cowan, 1979), such as the nucleus of the diagonal band of Broca. The septal nuclei is an evolutionary derivative of the hippocampus and the hypothalamus, and in the human brain is richly interconnected with both structures including the amygdala, and the substantia innomminata (SI) which is a major memory center, and which manufactures ACh--a transmitter directly implicated in memory (Gage et al., 1983; Olton, 1990). Andy and Stephan (1968) and Swanson and Cowan (1979) considered the bed nucleus of the stria terminals (which gives rise to a major pathway linking the septal nuclei, and amygdala and hypothalamus) as part of the septal nuclei, whereas others (Gloor, 2010) consider it to be part of the "extended amygdala." Likewise, some consider the nucleus accumbens as part of the septal nuclei, and others consider it part of the "extended amygdala;" i.e. the limbic striatum.
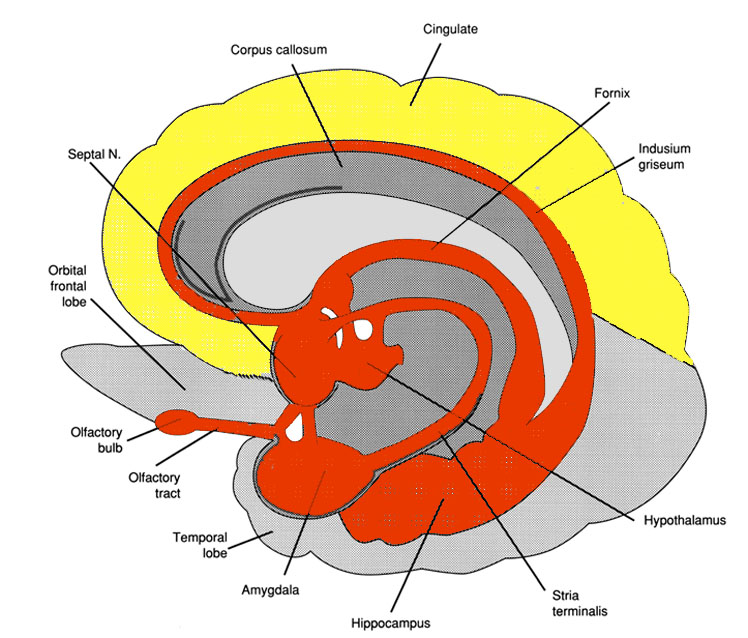
As noted the septal nuclei is massively interconnected with the hippocampus as well as with the entorhinal cortex (Swanson & Cowan, 1979) via a number of pathways, including the fornix. Directly implicating the septal nuclei in the memory functioning of the hippocampus is the finding that septal activation of this structure results in ACh secretion (Gage et al., 1983), whereas septal grafts into the hippocampus improves learning and memory (Gage et al., 2006). Conversely, lesions of the fimbria-fornix septal-hippocampal pathway results ACh depletion throughout the hippocampus (Gage et al., 1983; Olton, 1990), as well as loss of norepinephrine and serotonin coupled with memory loss (Olton, 1990).
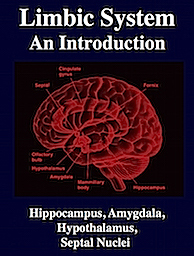
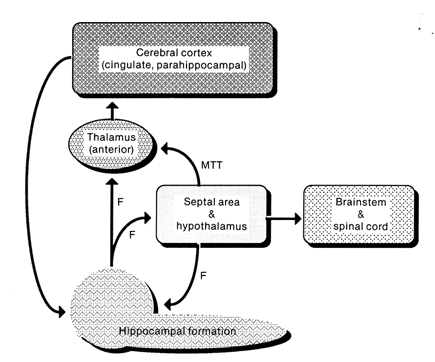
The septal nucleus in part regulate hippocampal memory-related activity not only by stimulating ACh and other neurotransmitter production (Gage et al., 1983, 2006), but as it provides excitatory input and inhibitory-GABAnergic-- especially from the medial septal nuclei which in general exerts inhibitory influences not only on the hippocampus but the amygdala and hypothalamus. In general, it is supposed that the excitatory-inhibitory influences on the hippocampus (like those on the amygdala and hypothalamus) serve to modulate activity and prevent extremes in arousal (Joseph, 1992a, 2012b, 2009d). This is accomplished in part not only through the interconnections maintained with the amygdala, hypothalamus and entorhinal cortex, but the brainstem reticular formation (Petsche et al., 1965)--with which the hippocampus is also connected directly and via the entorhinal cortex.
Septal influences on hippocampal/entorhinal arousal is also indicated by fluctuations in rhythmic slow activity (theta), which is generated by both the hippocampus and entorhinal cortex (Alonso & Garcia-Austt, 2007). As detailed in chapter 14, theta is an indication of hippocampal arousal (Green & Arduini, 1954; Petsche et al., 1965; Vanderwolf, 1992) and is associated with learning and memory (O'Keefe & Nadel, 2008). Theta is a robust electrophysiological phenomenon which has been found in the hippocampus of most species studied, including monkeys (Stewart & Fxx, 1990) and humans (Sano et al., 1970); though in primates it seems to differ from the theta rhythm of non-primates (see Gloor, 2010).
O'Keefe and Nadel (2008) believe that theta plays an important role in creating the spatial maps that are maintained by hippocampal "place" neurons; i.e. pyramidal neurons which are attuned to specific environmental features and landmarks and the animals place in that environment as they move about. Moreover, long term potentiation (LTP) which is associated with learning and memory, is generated in those neurons demonstrating theta or activity that is at the "theta frequency" (Staubli & Lynch, 2007).
Neurons of the septal nucleus which innervate the hippocampus fluctuate in activity in parallel with changes in the theta rhythm (Petsche et al., 1965), whereas septal lesions abolish hippocampal theta (Green & Arduini, 1954). It has long been believed that septal neurons act as an interface between the reticular formation and the hippocampus (Petsche et al., 1965) and in conjunction with its connections with the amygdala and hypothalamus, therefore modulate hippocampal arousal as well as learning and memory.
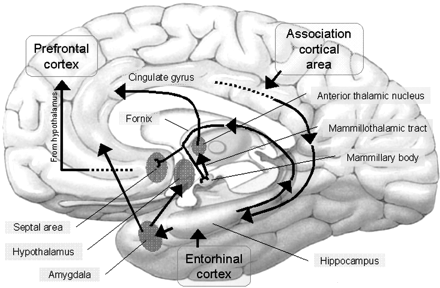
HIPPOCAMPAL & AMYGDALOID INTERACTIONS: MEMORY
It has been argued that significant impairments involving short-term memory and motor learning, cannot be produced by lesions supposedly restricted to the hippocampus (Horel, 2008; see also commentary in Eichenbaum et al. 2014); though in fact it is impossible to create such "restricted" lesions. Nevetheless, ignoring for the moment that inconvenient fact, in some instances with supposed restricted lesions, good recall of new information is possible for at least several minutes (Horel, 2008; Penfield & Milner, 1958; Squire 1992).
Moreover, there is considerable evidence which strongly suggests that the hippocampus plays an interdependent role with the amygdala in regard to memory (Gloor 1992, 2010; Halgren 1992; Kesner & Andrus, 2002; Mishkin, 2008; Murray 1992; Sarter & Markowitsch, 2005); particularly in that they are richly interconnected, merge at the uncus, and exert mutual excitatory influences on one another. For example, it appears that the amygdala is responsible for storing the emotional aspects and personal reactions to events in memory, whereas the hippocampus acts to store the cognitive, visual, and contextual variables (chapter 14) whereas that the amygdala activates the hippocampus by providing excitatory input (Gloor, 1955, 2010).
Specifically, the amygdala plays a particularly important role in memory and learning when activities are related to reward and emotional arousal (Gaffan 1992; Gloor 1992, 2010; Halgren 1992; LeDoux 1992, 2012; Kesner 1992; Rolls 1992; Sarter & Markowitsch, 2005). Thus, if some event is associated with positive or negative emotional states it is more likely to be learned and remembered.
The amygdala becomes particularly active when recalling personal and emotional memories (Halgren, 1992; Heath, 2015; Penfield & Perot, 1963), and in response to cognitive and context determined stimuli regardless of their specific emotional qualities (Halgren, 1992). However, once these emotional memories are formed, it sometimes requires the specific emotional or associated visual context to trigger their recall (Rolls, 1992; Halgren, 1992). If those cues are not provided or ceased to be available, the original memory may not be triggered and may appear to be forgotten or repressed. However, even emotional context can trigger memory (see also Halgren, 1992) in the absence of specific cognitive cues.
Similarly, it is also possible for emotional and non-emotional memories to be activated in the absence of active search and retrieval, and thus without hippocampal or frontal lobe participation. Recognition memory which may be triggered by contextual or emotional cues. Indeed, there are a small group of neurons in the amygdala, as well as a larger group in the inferior temporal lobe which are involved in recognition memory (Murray, 1992; Rolls, 1992). Because of amygdaloid sensitivity to visual and emotional cues, even long forgotten memories may be evoked via recognition, even when search and retrieval repeatedly fail to activate the relevant memory store.
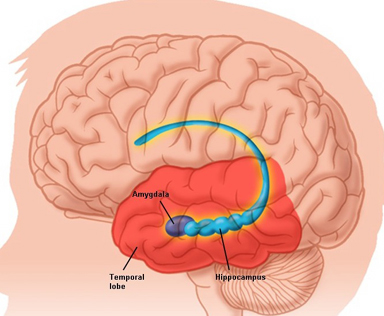
According to Gloor (1992), "a perceptual experience similar to a previous one can through activation of the isocortical population involved in the original experience recreate the entire matrix which corresponds to it and call forth the memory of the original event and an appropriate affective response through the activation of amygdaloid neurons." This can occur "at a relatively non-cognitive (affective) level, and thus lead to full or partial recall of the original perceptual message associated with the appropriate affect."
In this regard, it appears that the amygdala is responsible for emotional memory formation whereas the hippocampus is concerned with storing verbal-visual-spatial and contextual details in memory. Thus, in rats and primates damage to the hippocampus can impair retention of context, and contextual fear conditioning, but it has no effect on the retention of the fear itself or the fear reaction to the original cue (Kim & Fanselow 1992; Phillips & LeDoux 1992, 2012; Rudy & Morledge 2014). In these instances, fear-memory is retained due to preservation of the amygdala. However, when both the amygdala and hippocampus are damaged, striking and profound disturbances in memory functioning result (Kesner & Andrus, 2002; Mishkin, 2008).
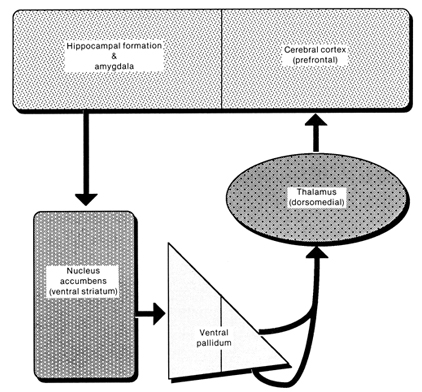
Therefore, the role of the amygdala in memory and learning seems to involve activities related to reward, orientation, and attention, as well as emotional arousal and social-emotional recognition (Gloor, 1992, 2010; Rolls, 1992; Sarter & Markowitsch, 2005). If some event is associated with positive or negative emotional states it is more likely to be learned and remembered. That is, reward increases the probability of attention being paid to a particular stimulus or consequence as a function of its association with reinforcement (Gaffan 1992; Douglas, 1967; Kesner & Andrus, 2002).
Moreover, the amygdala appears to reinforce and maintain hippocampal activity via the identification of motivationally significant information and the generation of pleasurable rewards (through action on the lateral hypothalamus). However, the amygdala and hippocampus act differentially in regard to the effects of positive vs. negative reinforcement on learning and memory, particularly when highly stressed or repetitively aroused in a negative fashion. For example, whereas the hippocampus produces theta in response to noxious stimuli the amygdala increases its activity following the reception of a reward (Norton, 1970).
In contract, right temporal destruction typically produces deficits involving visual memory, such as the learning and recall of geometic patterns, visual or tactile mazes, locations, objects, emotional sounds, or human faces (Corkin, 1965; Milner, 1965; Nunn et al., 2009; Kimura, 1963). Right sided damage also disrupts the ability to recognize (via recall) olfactory stimuli (Rausch et al. 1977), or recall emotional passages or personal memories (Cimino et al., 1991; Wechsler, 1973).
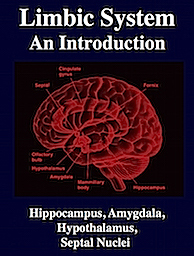
It appears, therefore, that the left amygdala and hippocampus are highly involved in processing and/or attending to verbal information, whereas the right amygdala/hippocampus is more involved in the learning, memory and recollection of non-verbal, visual-spatial, environmental, emotional, motivational, tactile, olfactory, and facial information. These issues and the differing roles of these nuclei in memory formation, as well as amnesia and repression will be discussed in greater detail in chapters 29, 30.
AMYGDALA & PLEASURE
The amygdala maintains a functionally interdependent relationship with the hypothalamus in regard to emotional, sexual, autonomic, consumatory and motivational concerns. It is able to modulate and even control rudimentary emotional forces governed by the hypothalamic nucleus. However, the amygdala also acts at the behest of hypothalamically induced drives. For example, if certain nutritional requirements need to be meet, the hypothalamus signals the amygdala which then surveys the external environment for something good to eat (Joseph, 2002, 1992a). On the other hard, if the amygdala via environmental surveillance were to discover a potentially threatening stimulus, it acts to excite and drive the hypothalamus as well as the basal ganglia so that the organism is mobilized to take appropriate action.
When the hypothalamus is activated by the amygdala, instead of responding in an on/off manner, cellular activity continues for an appreciably longer time period (Dreifuss et. al., 1968). The amygdala can tap into the reservoir of emotional energy mediated by the hypothalamus so that certain ends may be attained (Joseph, 2002, 1992a)
The amygdal-hippocampal complex, particularly that of the right hemisphere, is very important in the production and recollection of non-linguistic and verbal-emotional images associated with past experience. In fact direct electrical stimulation of the temporal lobes, hippocampus and particularly the amygdala (Gloor, 1990, 2010) not only results in the recollection of images, but in the creation of fully formed visual and auditory hallucinations (Gloor 1992, 2010; Halgren 1992; Halgren et al., 2008; Horowitz et al., 1968; Malh et al., 2015; Penfield & Perot, 1963), as well as feelings of familiarity (e.g. deja vus).
Indeed, it has long been know that tumors invading specific regions of the brain can trigger the formation of hallucinations which range from the simple (flashing lights) to the complex. The most complex forms of hallucination, however, are associated with tumors within the most anterior portion of the temporal lobe (Critchley, 1939; Gibbs, 1951; Gloor 1992, 2010; Halgren 1992; Horowitz et al. 1968; Tarachow, 1941); i.e. the region containing the amygdala and anterior hippocampus.
Similarly, electrical stimulation of the anterior lateral temporal cortical surface results in visual hallucinations of people, objects, faces, and various sounds (Gloor 1992, 2010; Halgren 1992; Horowitz et al., 1968)--particularly the right temporal lobe (Halgren et al. 2008). Depth electrode stimulation and thus direct activation of the amygdala and/or hippocampus is especially effective.
For example, stimulation of the right amygdala produces complex visual hallucinations, body sensations, deja vu, illusions, as well as gustatory and alimentary experiences (Weingarten et al. 1977), whereas Freeman and Williams (1963) have reported that the surgical removal of the right amygdala in one patient abolished hallucinations. Stimulation of the right hippocampus has also been associated with the production of memory- and dream-like hallucinations (Halgren et al. 2008; Horowitz et al. 1968).
The amygdala also becomes activated in response to bizarre stimuli (Halgren, 1992). Conversely, if activated to an abnormal degree, it may in turn produce bizarre memories and abnormal perceptual experiences. In fact, the amygdala contributes in large part to the production of very sexual as well as bizarre, unusual and fearful memories and mental phenomenon including dissociative states, feelings of depersonalization, and hallucinogenic and dream-like recollections (Bear, 1979; Gloor, 2006, 1992, 2010; Horowitz et al. 1968; Mesulam, 1981; Penfield & Perot, 1963; Weingarten et al. 1977; Williams, 1956). In addition, sexual feelings and related activity and behavior are often evoked by amygdala stimulation and temporal lobe seizures (Halgren, 1992; Jacome, et al. 1980; Gloor, 2006, 2010; Remillard, et al. 1983; Robinson & Mishkin, 1968; Shealy & Peele, 1957), including memories of sexual intercourse (Gloor 1990) or severe emotional trauma and abuse (Gloor, 2010).
Moreover, intense activation of the temporal lobe and amygdala has been reported to give rise to a host of sexual, religious and spiritual experiences; and chronic hyperstimulation (i.e. seizure activity) can induce some individuals to become hyper-religious or visualize and experience ghosts, demons, angels, and even God, as well as claim demonic and angelic possession or the sensation of having left their body (Bear 1979; Gloor 2006, 1992; Horowitz, Adams & Rutkin 1968; MacLean 1990; Mesulam 1981; Penfield & Perot 1963; Schenk, & Bear 1981; Weingarten, et al. 1977; Williams 1956).
LSD.
As is well known, LSD can elicit profound hallucinations involving all spheres of experience. Following the administration of LSD high amplitude slow waves (theta) and bursts of paroxysmal spike discharges occurs in the hippocampus and amygdala (Chapman & Walter, 1965; Chapman et al. 1963), but with little cortical abnormal activity. In both humans and chimps, when the temporal lobes, amygdala and hippocampus are removed, LSD ceased to produce hallucinatory phenomena (Baldwin et al. 2009; Serafintides, 1965). Moreover, LSD induced hallucinations are significantly reduced when the right vs. left temporal lobe has been surgically ablated (Serafintides, 1965).
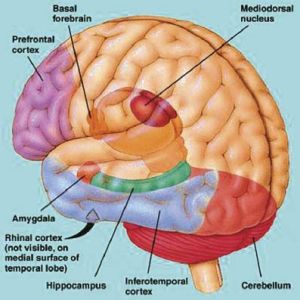
Overall, it appears that the amygdala, hippocampus, and the neocortex of the temporal lobe are highly interactionally involved in the production of hallucinatory experiences. Presumably, it is the neocortex of the temporal lobe which acts to interpret this material (Penfield & Perot, 1963) as perceptual phenomena. Indeed, it is the interrelated activity of the temporal lobes, hippocampus and amygdala which not only produce memories and hallucinations, but dreams. In fact, the amygdalas involvement in all aspects of emotion and sexual functioning, including associated memories, the production of overwhelming fear as well as bizarre and dream-like mental phenomenon, may well account for why this type of unusual stimuli, including personal and innocuous memories also appears in dreams.
The Right Hemisphere & Dreams.
There have been reports of patients with right cerebral damage, hypoplasia and abnormalities in the corpus callosum who have ceased dreaming altogether, suffer a loss of hypnogic imagery or tend to dream only in words (Botez et al. 2005; Humphrey & Zangwill, 1951; Kerr & Foulkes, 1981; Murri et al. 1984). However, there have also been some report that when the left hemisphere has been damaged, particularly the posterior portions (i.e. aphasic patients), the ability to verbally report and recall dreams also is greatly attenuated (e.g., Murri et al. 1984). Of course, aphasics have difficulty describing much of anything, let alone their dreams.
Electrophysiologically the right hemisphere also becomes highly active during REM, whereas, conversely, the left brain becomes more active during N-REM (Goldstein et al. 1972; Hodoba, 2006). Similarly, measurements of cerebral blood flow have shown an increase in the right temporal regions during REM sleep and in subjects who upon wakening report visual, hypnagogic, hallucinatory and auditory dreaming (Meyer et al. 2007). Interestingly, abnormal and enhanced activity in the right temporal and temporal-occipital area acts to increase dreaming and REM sleep for an atypically long time period (Hodoba, 2006). Hence, it appears that there is a specific complementary relationship between REM sleep and right temporal electrophysiological activity.
Interestingly, daydreams appear to follow the same 90-120 minute cycle that characterize the fluctuation between REM and NREM periods, as well as fluctuations in mental capabilities associated with the right and left hemisphere (Broughton, 2002; Kripke & Sonneschein 1973). That is, the cerebral hemisphere tend to oscillate in activity every 90-120 minutes -- a cycle which appears to correspond to the REM-NREM cycle and the appearance of day and night dreams.
Forgotten Dreams.
Most individuals, however, have difficulty recalling their dreams. This may seem paradoxical considering that hippocampal theta is being produced. However, this is theta punctuated by high levels of desychronized activity, which is not conducive to learning. In this regard, theta activity may represent the reverberating activity of neural circuits formed during the day, such that the residue of day time memories come to be inserted into the dream. Conversely, due to the high level of desychronization occuring in the hippocampus (as it is so highly aroused), although it contributes images and the days memories, it does not participate in storing these dream-like experiences into memory.
Consider the results from temporal lobe, amygdala, and hippocampal electrical stimulation on memory recall and the production of hallucinations. Although personal memories are often activated at low intensities of stimulation (memories which are verified not only by the patient but family), if stimulation is sufficiently intense, the memory instead will become dreamlike and populated by hallucinated and cartoon like characters (Halgren, et al. 2008). That is, at low levels of stimulation memories are triggered but these memories become increasingly dream-like with high levels of activity. Moreover, once these high levels of stimulation are terminated, patients soon become verbally amnesic and fail to verbally recall having had these experiences (Gloor, 1992; Horowitz, et al. 1968). However, these memories can be later recalled if subjects are provided with specific contextual cues (Horowitz, et al. 1968). The same can occur during the course of the day when a fragment of a conversation, or some other experience, suddenly triggers the recall of a dream from the previous night which had otherwise been completely forgotten. Presumably it had seemingly been forgotten because the hippocampus did not participate in their storage and thus could not assist in their retrieval (see chapters 29, 20).
There is also some evidence to suggest that different regions of the hippocampus show different levels of arousal during paradoxical sleep. For example, it appears that the posterior hippocampus becomes activated during paradoxical sleep and shows theta activity, whereas the more anterior portions become inhibited (Olmstead et al. 1973). As the anterior portions are more involved in new learning (at least in humans), whereas the posterior hippocampus is more concerned with old and well established memories, this would suggest that the posterior hippocampus is contributing older or already established memories to the content of the dream--which explains why theta, which is associated with learning and memory, is also produced during the dream--that is, it is replaying various fragmentary memories. Conversely, the inhibition of the anterior region would prevent this dream material from becoming re-memorized.
DREAMS & INFANCY
In the newborn, and up until approximately 6-9 months, there are two distinct stages of sleep which correspond to REM and N-REM periods demonstrated by adults (Berg & Berg, 2008; Dreyfus-Brisac & Monod, 1975; Parmelee et al. 1967). Among infants, however, REM occur during wakefulness as well as during sleep. In fact, REM can be observed when the eyes are open, when the infant is crying, fussing, eating, or sucking (Emde & Metcalf, 1970). Moreover, REM is also observed to occur within a few moments after an infant begins to engage in nutritional sucking and appears identical to that which occurs during sleep (Emde & Metcalf, 1970).
The production of REM during waking in some respects seems paradoxical. Nevertheless, it might be safe to assume that like an adult, when the infant is in REM, he or she is dreaming, or at least, in a dream-like state. Possibly, this state corresponds to what Freud has described as the Primary Process. That is, when produced when the infant is crying or fussing, it is dreaming of whatever relief it seeks. Correspondingly, REM which occurs while eating or sucking may have to do with the limbic structures which are involved not only in the production of dream-like activity, but the identification, learning and retention of motivationally significant information (i.e. the amygdala and hippocampus).
Presumably this relationship is a consequence of REM as well as eating and sucking being mediated, in part, by the amygdala as well as other limbic nuclei, which are also concerned with forming motivationally significant memories. Hence, when hungry, the hypothalamus becomes aroused which activates the amygdala which is responsible for the performing environmental surveillance so as to attend, orient to, identify and approach motivationally significant stimuli and eat. However, because the infants brain is so immature and as its resources for meeting its limbic needs are quite rudimentary, under certain conditions prolonged hypothalamus induced amygdala activation results in the formation and recall of relevant memories which may be experienced as hallucinations of the desired object. That is, previously formed neural networks become activated and the infant begins to dream and hallucinate food and will then suck and smack its lips as if eating or sucking when it is awake, in REM, and there is no food present.
THE PRIMARY PROCESS
The hypothalamus, our exceedingly ancient and primitive Id, has an eye that only sees inward. It can tell if the body needs nourishment but cannot determine what might be good to eat. It can feel thirst, but has no way of slacking this desire. The hypothalamus can only say: "I want", "I need", and can only signal pleasure and displeasure. However, being the seat of pleasure, the hypothalamus can be exceedingly gracious in rewarding the organism when its needs are met. Conversely, when its needs go unmet it can respond not only with displeasure and feelings of aversion, but with undirected fury and rage. It can cause the organism to cry out.
Nevertheless, the cry does not produce the immediately desire relief or reduction in tension. There is thus a pressure on the limbic system and the organism to engage in environmental surveillance so as to meet the needs monitored by the hypothalamus.
Over the course of the first months of life, as the amygdala and then hippocampus develop, the organism begins to develop an eye that not only sees outward, but which can register and recall events, objects, people, etc., associated with tension reduction, pleasure and the satiaty of the infants internal needs (e.g. the taste, smell, feeling of mother's breast and milk, the experience of sucking and relief, etc.). This is called learning.
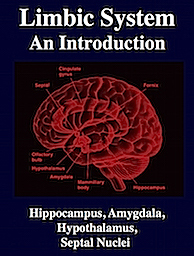
With the maturation of these two limbic nuclei the infant is increasingly able to differentiate what occurs in the external environment based on hypothalamically monitored needs and the emotional/motivational significance of that which is experienced. The infant can now orient, selectively attend, determine what brings satisfaction, and store this information in memory.
PRIMARY IMAGERY
Although admittedly we have no direct knowledge as to the psychic interactions in the neonate, it does seem reasonable to assume that as the neocortex and underlying structures and fiber pathways mature, neural "prgroams" are formed which correspond to the repeated registration of experiences which are deemed significant (e.g. pleasurable). That is, neural pathways which are repetitively fired, deactivated or activated in response to specific sensory and affective activities and experiences, become associated with that activity, such that an associated neural circuit is formed (chapter 14); i.e. a memory is created. Eventually, if this circuit is reactivated, the "learned" pattern is reexperienced; i.e. the organism remembers.
Thus, infants as young as 2 days of age can learn to suck at the mere sight of a bottle (see Piaget, 1954) and in order to receive milk as a reinforcement, infants can even modify their sucking response (Sameroff & Cameron, 1979). Hence, they are susceptible to classical conditioning (Sameroff & Cavanagh, 1979), although the possibility of operant conditioning has not been established. Nevertheless, the fact that they can recognize the bottle and suck (as well as cry and shed tears) indicates that various regions of the limbic system, especially that of the amygdala is functional and that learning and the creation of specific, context specific neural circuits have been formed very early in life.
Thus, when the amygdala/hippocampus are stimulated by a hungry hypothalamus, the events and images associated with past experiences of pleasure can not only be searched out externally, but recalled in imaginal form. For example, as an infant experiences hunger and stomach contractions as well as it own cries of displeasure, these states become associated with the sound, smell, taste, etc. of mother and her associated movement and other stimuli which accompany being fed (cf Piaget, 1952, pp. 37, 407-408). Repetitively experienced, the sequence from hunger to satiety evokes and becomes associated with the activation of certain neural pathways and the creation of a specific neural network subserving that memory (chapter 14).
Eventually, when the infant becomes hungry, if prolonged there is the possibility that the entire neural sequence associated with hunger and feeding (i.e. hunger, mother, food, satiaty), may become involuntarily triggered and activated (via association) such that an "image" of being fed is experienced. The activation of these rudimentary and infantile memory-images is probably what consititutes, at least in part, the primary process.
Behaviorally this is manifested by REM and via sucking and tongue movements as if eating, when in fact there is no food present (cf, Piaget, 1952). That is, when hungry, the infant will begin to cry, rapid eye movement (REM) might be observed, and then the infant will stop crying and smack its lips and make sucking movement (mediated by the amygdala) as if it were being fed. The infant experiences the experience of being fed in the form of a dream (Joseph, 2002) or hallucination, although it is awake.
In that the brain of the human infant is quite immature for in fact several years, which in turn restricts information reception and processing (chapters 23-28), and given the limited amount of reality contact infants are able to achieve, these rudimentary memories and images (even when occurring during waking, i.e. REM), are probably indistinguishable from actual experience simply because they are experience.
Like a dream, when replayed, the infant presumably reexperiences to some degree the sensations, emotions, etc., originally linked to tension reduction. Thus, the young infant, as yet unable to distinguish between representation and reality, responds to the image as reality (Freud, 1900, 1911), even while awake--as manifested by REM. When hunger is prolonged the association linked to feeding are triggered and for a brief time period the infant behaves as if its hunger has been sated. Reality is replaced by an image, or rather, a "dream". This is the primary process.
Since the hypothalamus (which monitors internal homeostasis) is not conscious that the dream images experienced are not real, it initially accepts the memory/dream images transmitted from the amygdala and hippocampus and ceases to cry, i.e. it responds to the imagined sources of nourishment just as it responds to a cue-tone associated with a food reward (Nakamuar & Ono, 2006; Ono et al., 1980). However, the hypothalamus is not long fooled, for the primary process does not offer effective long lasting relief from tension. As the pain of hunger remains and increases, limbic activity is increased, and the image falls away to be replaced by a cry of hunger (Joseph, 2002). The amygdala and hippocampus are thus forced to renew their surveillance of the environment in search of sources of tension reduction. Cognitive development is thus promoted.
"Whatever was thought of (desired) was simply imagined in an hallucinatory form, as still happens today with our dream-thoughts every night. This attempt at satisfaction by means of hallucination was abandoned only in consequence of the absence of the expected gratification, because of the disappointment experienced. Instead, the mental apparatus had to decide to form a conception of the real circumstances in the outer world and to exert itself to alter them...The increased significance of external reality heightened the significance also of the sense-organs directed towards the outer word, and of the consciousness attached to them; the later now learned to comprehend the qualities of sense in addition to the qualities of pleasure and "pain" which hitherto had alone been of interest to it. A special function was instituted which had periodically to search the outer word in order that its data might be already familiar if an urgent need should arise; this function was attention. Its activity meets the sense-impressions halfway, instead of awaiting their appearance. At the same time there was probably introduced a system of notation, whose task was to deposit the results of this periodical activity of consciousness--a part of that which we call memory" (Freud, 1911, pp. 410-411).
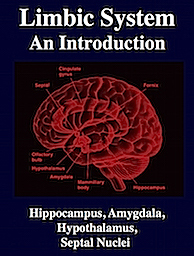