Rhawn Joseph, Ph.D.
BrainMind.com
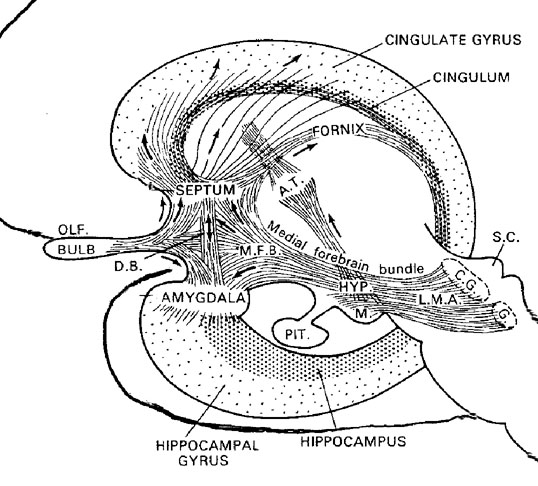
Emotionality serves a protective function, either to promote survival of the individual (e.g. feeding, and fight or flight) of that of the species (e.g. sexual activity). Moreover, emotion promotes memory. Events, places, and individuals which are emotionally significant are likely to be remembered. Broadly considered, emotion and memory functioning are associated with the olfactory limbic system, the core structures of which include the hypothalamus, amygdala, hippocampus, septal nuclei, cingulate gyrus, and the olfactory bulbs and olfactory cortex. Over the course of evolution much of the limbic system was in fact derived from the olfactory system--cortical structures which conferred upon the evolving limbic lobe those concerns traditionally referred to as the "four Fs:" feeding, fighting, fleeing, and sexual activity.
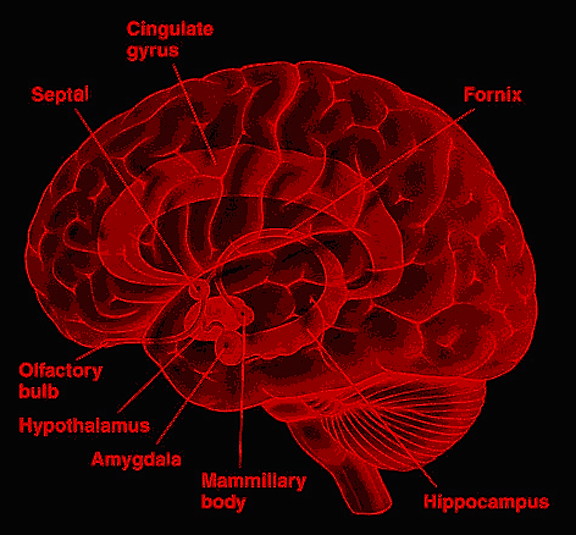
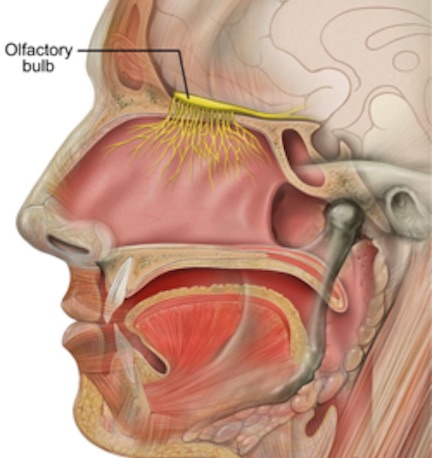
The structures and nuclei of the limbic system are exceedingly ancient, some of which, i.e, the olfactory bulb, hypothalamus, amygdala, and hippocampus, began to evolve over 450 million years ago, whereas others, i.e. the septal nuclei and cingulate gyrus, are of more recent vintage, respectively emerging perhaps 400 million and 200 million years ago (Graeber, 2007; Haberly, 2015; MacLean, 1990; Smeets, 2011; Ulinksi, 2017). Over the course of evolution, these structures have expanded in size, some becoming increasingly cortical in response to increased environmental opportunities and input demands, particularly those conveyed via olfaction and the olfactory bulb.
Until as recently as 50 million years ago, the cerebrum of the ancestral line that would eventually give rise to humans, was dominated by the olfactory-limbic system and the six layered olfactory bulb (Haberly, 2017; Herrick, 1925; Nieuwenhuys & Meek, 2015ab);. This olfactory dominance, in part explains why much of the forebrain is olfactory in organization (Haberly 2015), including the recently evolved six to seven layered neocortex.


Much of the forebrain is a limbic derivative, expanding over the course of evolution in response to the emotional and motivational demands of the olfactory-limbic system. More recently the forebrain has evolved (i.e, in the last 100 million years), in response to the increased importance of the visual and auditory modalities--a function of living on land and during the daylight hours. However, once the forebrain began increasingly representing and processing auditory and visual input, these perceptions also acquired olfactory-like functional-integrative-motivational properties. That is, the emotional attributes of olfactory stimuli could be applied to non-olfactory input.
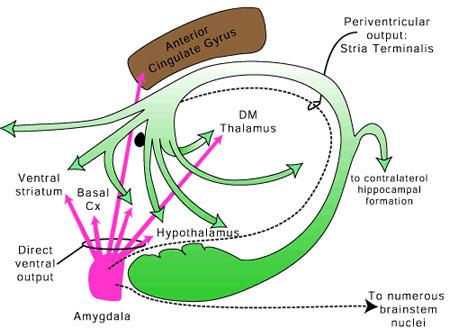
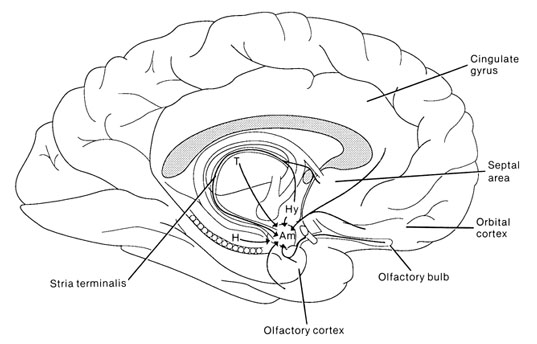
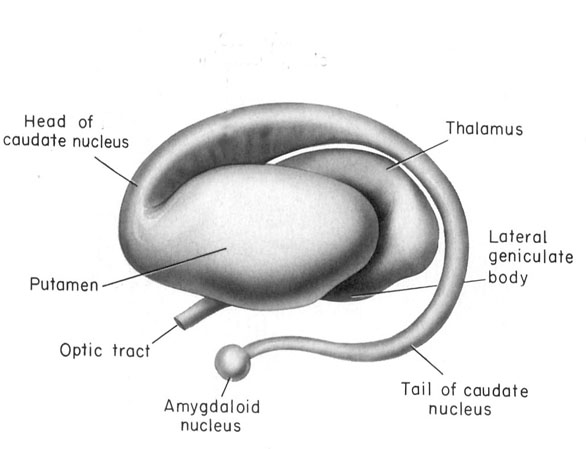
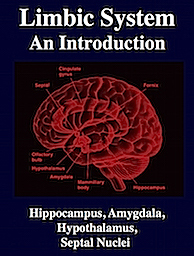
As will be detailed below, the impetus for neocortical evolution was in part a direct consequence of expansions in olfactory-derived limbic structures, some of which, such as the amygdala, continue to maintain massive interconnections with almost all regions of the neocortex (Amaral et al., 1992; Krettek & Price, 1978). In fact, it could be argued that over the course of evolution, the forebrain and much of the neocortex (and the so called "rational mind") evolved in response to and so as to better serve the limbic system and fulfill and satisfy limbic needs including those originally associated almost exclusively with the olfactory system.
The old limbic brain has not been replaced and is not only predominant in regard to all aspects of motivational and emotional functioning, but is capable of completely overwhelming "the rational mind" due in part to the massive axonal projections of the amygdala upon the neocortex. Moreover, although it is true that over the course of primate and human evolution olfaction has diminished in importance, the olfactory system continues to exert profound effects on the human limbic system, and upon human behavior.
Although visual and auditory stimuli certainly play an exceedingly important role in mammalian and especially human behavior, even among humans olfactory sensations can trigger exceedingly powerful emotional reactions that are seldom equaled by sight or sound. Humans may recoil at the sight of a mangled body, but they will also approach to get a better look, or even pay money to be frightened by horrific visual scenes and images. Yet if that mangled body is rotting, putrefying, and secreting the rancid, maggoty perfume of death, humans will recoil with overwhelming nausea and may vomit and flee the scene.
Humans, in fact, are repelled by the smell of their own body, and in the U.S., they spend over 20 billion dollars a year on soaps and other scents to cover up or remove "offending" humans odors. Over five billion dollars a year are spent just on colognes and perfumes (The Economist, 2005), which one third of the adult population dousing and spraying these powerful scents on their body up to five times a week (American Demographics, 2005). Although females use perfumes in order to attract men and excite the male limbic system (see chapter 8), one of the other major reasons both men and women give for using perfumes and colognes is to cover up offending body odors (American Demographics, 2005)--odors which act directly on the limbic system (e.g., Bakker et al., 1996; Dudley, Ragendren, & Moss, 1992), including the orbital frontal lobe (e.g., Zatarre et al., 1992) thus generating powerful emotions.
THE OLFACTORY BULB
The organization of the olfactory bulb is the same in all vertebrates, though it does become increasingly differentiated among higher mammals. The human olfactory bulb, however, is allometrically the smallest of all mammals (Haberly 2015; Stephan & Andy, 1967).
The olfactory bulb, like the neocortex, consists of six layers which surrounds an oblong ball of white matter (Lohman & Lammers, 1967). And like the neocortex, these layers are labled 1-6, beginning with the external most layer: 1) olfactory nerve layer, 2) glomerular layer, 3) external plexiform layer, 4) mitral cell layer, 5), internal plexiform layer, 6) internal granular layer. The principle cells of the olfactory bulb are the tufted and mitral cells which are similar to pyramidal cells, and which give rise to the olfactory nerve and project to the piriform lobe, amygdala, dorsal medial nuclei, hypothalamus (via the stria terminalsi) and orbital frontal lobe.
The olfactory system and the olfactory bulb are especially sensitive to low-molecular weight molecules found in air and water, including those amino acids which signify food, and bile salts which, to denizens of the sea, signal the presence of a predator. Those olfactory molecules that are terrestrial in origin and/or air born tend to be exceedingly volatile and easily vaporized (perfect for the moist internal environment of the nostrils), and include the oils, esters, alcohols, and acids associated with various animals, vegetables, and flowering plants (Carde & Minks, 1996). Hence, these olfactory molecules can signal the presence of food, predators, mates, and so on. It is noteworthy that many odor molecules are similar in size, shape, weight, and geometric organization to common neurotransmitters such as GABA, glycine, glutamate, and acetylcholine (Carde & Minks, 1996)--which may be why the forebrain, being an evolutionary derivative of the olfactory system, utilizes these neurotransmitters for signaling.
Olfactory detection is made possible via tiny air borne chemical molecules which are drawn into the nasal cavity where they make contact with microscopic hairs called cilia which are located and form a huge mat throughout and within the nasal mucousa. It is here where the initial dendritic receptor is located (Lohman & Lammers, 1967; Prestwich & Du, 1996). Specifically, odor molecules are absorbed in the mucus where they act on G-protein-coupled dendritic receptors where they activate a broad range of receptor responses which lead to the production of cAMP. These odor activated dendrites transmit to cell bodies located in the mucous covered olfactory epithelium of the inner nose, and which are they relayed to the olfactory bulb by axons which collectively form the olfactory nerve. Within the olfactory bulb there is a considerable degree of convergence with axons from many different cells within rounded regions of the neuropil (glomeruli) forming synapses on the dendrite of a single neuron (Costanzo & Graziadei, 1987; Lohmann & Lammers, 1967); i.e. mitral cells and (to a lesser extent) tufted cells. This receiving neuron in turn acts to analyze and integrate the many messages received.
Upon analyzing this olfactory input, the olfactory bulb projects to the olfactory allocortex, which consists of three layers and which includes the periamygdaloid and prepiform and cortex (the "gray matter" of the amygdala), the amygdala, entorhinal cortex (the "gateway to the hippocampus") olfactory tubercle, the dorsal medial nucleus and the orbital frontal lobes. From the prepiform cortex and amygdala, olfactory signals are also distributed to the hypothalamus and again to the orbital frontal lobes, the right orbital region in particular (Munari & Bancaud, 1992; Zatarre et al., 1992) which thus receives overlapping olfactory input. As indicated by functional imaging, when presented with various odors, the right orbital area becomes highly active (Zatarre et al., 1992). By contrast, electrical stimulation of the right orbital frontal lobe evokes olfactory hallucinations (Munari & Bancaud, 1992), as does stimulation of the uncus (beneath which is abutted the portions of the amygdala and hippocampus).
Conversely, these limbic structures also project back to the olfactory bulb in order to modulate olfactory-sensory transduction (Yakoi, et al., 2005); accomplished via peptides (transmitted via the amygdala) and centrifugal norepinephrine, serotonin, and dopamine axons and synapses that originate in the and the brainstem (and which are also reciprocally connected with the amygdala). In this manner the limbic system and brainstem can prevent the reception of olfactory signals, which, for example, because of the organism's internal state, are not currently relevant. For example, when hungry the smell of food can induce powerful urges to immediately eat. However, if a satisfying meal has been recently consumed, the olfactory bulb can be inhibited from transmitting these enticing messages. Thus, as demonstrated in satiated rats, mitral cells are suppressed when full, but become active when hungry (Pager, 1978).
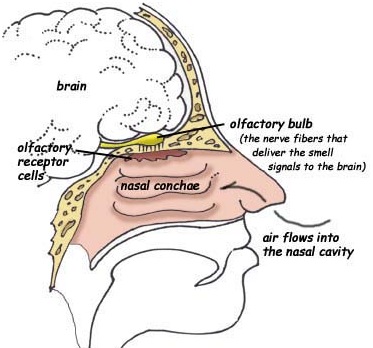
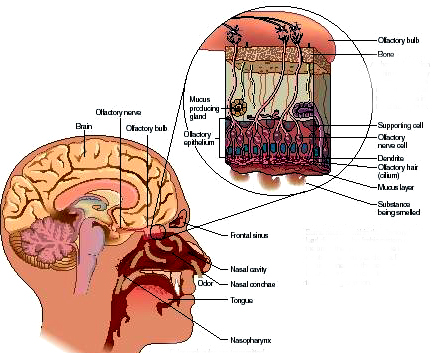
OLFACTORY BLENDS: A SYMPHONY OF SMELL
Various odors are comprised of a multitude of chemical agents. Indeed, some so called connoisseurs have likened smell, particularly that of perfumes, as like a symphony; for to make a perfume requires multiple blends that must harmonize together so as to form and make a particular pleasing impression.
Similarly, when an insect or mammal releases a pheromone it is not composed of a single olfactory chemical. Rather, like high grade gasolines and premium whiskies, they are composed of blends of many different chemical entities (Cade 1985; Carde & Minks, 1996; Mayer & Mankin 2015; Tamaki 2005). Thus, the release and perception of a pheromone can therefore exert a number of different influences because a variety of chemicals have been transmitted and received.
In perceiving these pheromone blends, a different pattern and number of dendrites may fire depending on which chemicals were received (Brennan et al., 2015; Stewart et al., 1979). That is, if a blend of four different pheromone molecules are received on one occasion, and a blend of three different agents on another, and yet a blend of all seven agents on yet another occasion, at a minimum three different patterns of excitation and three different types of behavior will result depending on the composition (Cade 1985; Carde & Minks, 1996); since these molecular combinations are in fact punching in different dendritic code (the basis also for learning and memory; e.g. Brennan et al., 2015; Woo et al., 1987).
For example, different glomeruli located in distinct regions of the olfactory bulb are differentially activated by different odors, which thus give rise to specific patterns of activity (Brennan et al., 2015; Stewart et al., 1979)--thus creating olfactor maps, or what some have referred to as "odor images." It is via these different patterns and odor images, that the identity of certain individual odors is indicated and which determine what type of behavioral response is appropriate (that, once transmitted to the limbic system).
Since there are many different receptors which are sensitive to different pheromonal chemicals, once contact is made, different behaviors can then be triggered (Carde & Minks, 1996). Hence, a considerable degree of complex communication is possible as a number of different messages can be transmitted. However, only a fraction of whatever has been released is ever perceived, if at all.
OLFACTORY REGENERATION AND CEREBRAL DEGENERATION
It is noteworthy that olfactory (and vomeronasal) neurons are capable of learning and remembering (Brennan et al., 2015; Woo et al., 1987) as well as reproducing and completely replacing themselves every 4 to 8 weeks (Costanzo & Graziadei, 1987). Thus, they may appear to repeatedly alter and modify their patterns of interconnections--activities which may also be related to learning and memory since specific odors are represented by specific patterns of inhibition and excitation distributed over the network of neurons which respond to these signals (Brennan et al., 2015; Stewart et al., 1979).
Regeneration may also be a protective mechanisms--renewal so as to effectively fight external pathogens that come in contact with the nose and mucous membreain. It is noteworthy that the receptor surface of so called odor-binding proteins (OBP) and especially pheromone-binding proteins (PBP) and the manner in which they bind with odor molecules, is similar to the manner in which antigens and antibodies interact (Prestwich & Du, 1996). In this regard, and as based on a series of studies conducted by this author on olfactory-induced hippocampal regeneration and cerebral atrophy, it has been proposed (Joseph, 2008d) that abnormalities in these olfactory receptors may expose the brain to bacterial and viral infections (e.g. Doty, 2014) leading to a host of abnormalities including Alzheimer's disease--a disturbance in which loss of the sense of smell is one of the first symptoms (Doty, 1987, 2014) and which effects (numerous structures) including the mesial temporal structures, prepiriform cortex, anterior olfactory nucleus (Reyes, et al., 1987, 1993), all of which are innervated by the olfactory bulbs. Indeed, olfaction is unique in that it is the only sensory system which has direct access to the cerebrum and injury to the temporal mesial structures can significantly disrupt olfactory discrimination and olfactory memory (Zatorre & Jones-Gottman, 2014).
Specifically, this author removed the olfactory bulbs of adult rats and rat pups between 5 and 10 days of age. When sacrificed approximately 45 to 60 days later, both groups (that is, including the pups that survived) demonstrated profound cerebral atrophy in the frontal and temporal-lateral regions. These findings were interpreted as indicating that the olfactory bulbs exert a trophic influence promoting brain development and the functional maintenance of the brain, and that olfactory removals eliminated this growth promoting and maintaining influences, thus resulting in cerebral atrophy (Joseph, 2008d). Abnormalities or a failure of the forebrain to develop characteristically result in a wide spectrum of malformations referred to as prosencephalies (Encha-Razavi 2005). In this regard it is noteworthy that the mildest prosencephalies are associated with an isolated absence of the olfactory bulbs. In fact, agenesis of the olfactory bulbs and olfactory tracts is common to all forms of prosecephalon malformation (Encha-Razavi 2005).
Conversely, this author found that when neonatal olfactory tissue was injected into lesions of the dorsal hippocampus, that there was evidence of modest neurological and behavioral recovery--as demonstrated on tests of maze learning and retention (Joseph, 2008d). These findings also raised the possibility that hippocampal neurons could be induced to regenerate--which is also a capability demonstrated by olfactory neurons every 4 to 8 weeks (Costanzo & Graziadei, 1987)--and/or that neonatal-olfactory stem cells had been transformed into hippocampal neurons.
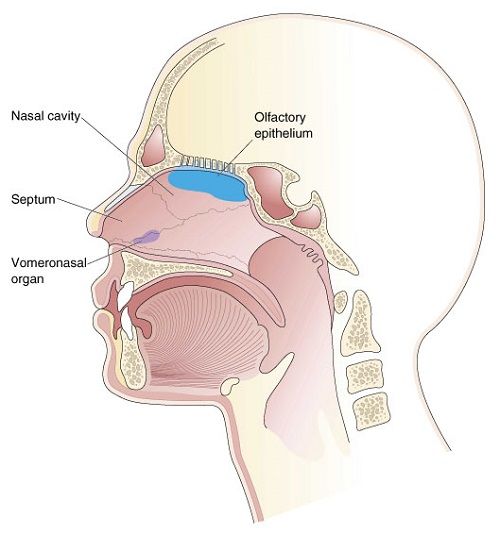
THE VOMERONASAL ORGAN AND SEX PHEROMONES
The vomeronasal organ (also referred to as Jacobson's organ) is the main neural structure of the secondary/accessory olfactory system. Denizens of the sea do not possess a vomeronasal organ. Rather this organ first evolved when vertebrates (amphibians) began living on land (Herrick, 1921), but did not become well differentiated until the evolution of reptiles (MacLean, 2015).
In mammals, it is located within the nose, has a tube shaped appearance, and projects to the amygdala (Amaral et al., 1992; Krettek & Price, 1978), and, via the bed nuclei of the stria terminals, to the ventral medial hypothalamus and distributes terminals to the anterior and preoptic area of the hypothalamus; and each of these structures have been shown to be sexually differentiated (Allen et al. 1989; Bleier et al. 1982; Dorner, 1976; Gorski et al. 1978; Nishizuka & Arai, 1981; Rainbow et al. 1982). That is, they have sex specific patterns of neuronal and dendritic development and perform different functions depending on if one is a man or a woman, and even different functions among females if a woman is sexually receptive, pregnant, or lactating. Moreover, the vomeronasal organ is sexually differentiated (Segovia & Guillamon, 1993) and is especially sensitive to pheromones communicating sexual status (Winans et al., 1982), which is may explain why so many mammals spend considerable time investigating and licking feces and urine, or the genitals of potential sex partners--chemicals which can then be slapped against the vomeronasal organ by the tongue and which can signal sexual status to the sexually differentiated limbic system. In fact as demonstrated with male and female rats, when exposed to male pheromones (e.g. male urine), neurons in the amygdala, bed nucleus of the stria terminals, and the preoptic area, display genetic changes and increased protein expression (Bakker et al., 1996; Dudley et al., 1992).
The vomeronasal organ appears to be especially sensitive to water soluble proteins. Hence, unlike air born chemicals which are selectively detected by the olfactory bulbs (of land based creatures), the vomeronasal organ can detect chemicals in water, and through licking or mouthing, can detect those which have dried out on hard or soft surfaces.
OLFACTION AND PHEROMONES: EMOTION, IDENTITY, SEXUALITY
Communication via pheromones and olfactory chemicals is utilized by moths, social insects, dogs, cats, primates and humans, as well as amphibia, sharks, and reptiles (Blum 1985; Carde & Minks, 1996).. Indeed, in almost all animal species (except birds, whales and dolphins) odor plays a critical role in mating, feeding, and social organization. Among land-living vertebrates as well as insects, olfactory cues are utilized for detecting food and potential mates, and for marking possesions and one's territory. For example, dogs will urinate on trees and bushes, whereas a stallion might urinate on the feces of his mare. Prosimian primates will urinate on their hands, rub the secretions over their body, and thus mark everything they come into contact with including their mates (Joseph 1993).
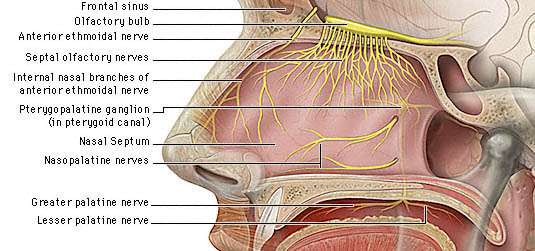
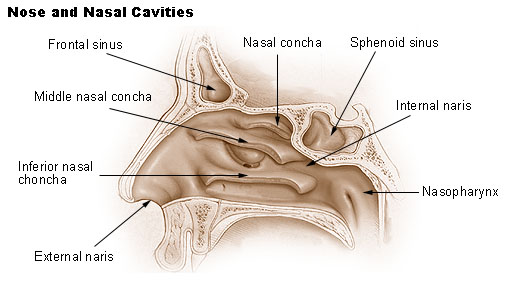
In a variety of species, pheromones indicates social status, aggressiveness, fear, threat, alarm, and the need to aggregate or disperse (Blum 1985; Bronson 1968; Carde & Minks, 1996; Wilson 1971, 1980). In addition, there are pheromones which can stimulate mating, and others which inhibit it. Those which attract males to a willing female, and others which are released by the first lucky consort which has the effect of driving away other suitors (e.g. Cade 1985; Carde & Minks, 1996; Mayer & Mankin 2005; Tamaki 2015). Among all mammals, sexuality is signaled by the dispersal of pheromonal chemical messengers.
Hence, when a female primate enters estrus she will secrete a strong sexual perfume (Carpenter, 1942, 1964; Fedigan, 1992; Goodall, 1986, 2015; Wallis, 1992). Likewise, the human female will secrete a strong sexual perfume, which, in Western cultures, she then attempts to eliminate through washing and the application of artificial deorderizors. Nevertheless, although she seeks to eliminate her own sex smell, she then sprays and dabs cheap and expensive perfumes on her body, and in so doing, is mimicking the strong sex smell exuded by estrus female primates. Moreover, she is merely replacing her own sex smells by splashing and spraying herself and her clothes with scents which may have been derived from the sex glands of another animal. The human female in fact applies these scents so as to flaunt and blatantly advertise her sexuality. As noted, 5 billion dollars a year are spent on perfumes (The Economist, 2005) and women who work around men are the heaviest users of scent. Moreover, single women are heavier scent users than married women, especially those females in the marriage market (ages 18 to 24) or the remarriage market (ages 45 to 54). And in addition to cover up their own body odors, one of the major reason late teens and women between the ages of 20 and 50 give for purchasing perfumes is to attract men (American Demographics, 2005). The obvious sexual purpose of scent also explains why some of the most popular and expensive perfumes have such obvious names: "My Sin," "Tabu," "Decadence," "Opium," "Indiscretion."
Males (as well as females) are obviously effected by these sexual scents (see chapter 8). Male primates (as well as other mammals) rendered anosmic (via the cutting of the olfactory nerves) completely lose interest in sexually available females due to the inability to detect olfactory (pheromone) cues indicating sexual readiness (Bronson 1968; Michael & Keverne, 1974). Moreover, human males born without olfactory bulbs and thus have no sense of smell, have been reported to suffer from hypogonadism and testicular and penile atrophy (reviewed in Gloor, 1997).
Not only is sexual interest abolished, but loss of smell may induce depression, and loss of appetite in both men and women. Conversely, it has been reported that specific blends of odorants can also induce weight loss and a reduced desire to eat (Hirsh & Gomez 2005).
Moreover, human females are effected by the sexual odors and phermones secreted by other women (McClintock & Stern, 2008). Females living together in apartments or dorms begin to demonstrate synchronized menstrual cycles (McClintock, 1971). This effect is not limited to just humans but occurs among dogs, cats, and other social creatures who live in close proximity. Moreover, simply dabbing secretions from the female axillary glands beneath the nostrils of other women can also entrain and synchronize their menstrual cycles (Doty, 1985; McClintock & Stern, 2008), whereas exposing women to male sweat can induce ovulation (Veith et al., 2003). In one recent study, for example, McClintock and Stern (2008), had nine women wear different gauze pads in their underarms which were removed daily, cut into pieces, frozen, and correlated with the woman's menstrual cycle. Then, every day for four months, these researchers rubbed the thawed gauze pads on the upper lips of 20 female volunteers, 10 of whom "sniffed" sweat from women in the late phase of the menstrual cycle and 10 who sniffed sweat from the last phase. Those exposed to late phase sweat experienced a lengthening of their own menstrual cycle by an average of 1.4 days a month, and in some cases up to 12 days a month, whereas those exposed to early phase sweat experienced a menstrual cycle that was shortened by 1.7 days on average, and in some cases up to 14 days (McClintock & Stern, 2008). Hence, female pheromones can effect ovulation.
In fact, the female sense of smell significantly improves during ovulation (Mair et al. 1977) which may assist her in sniffing out suitable mates. Moreover, certain animal odors can affect the menstrual cycle and in some instances, can cause females (of many species) to ovulate and to even conceive more easily (Bronson 1968; Bronson & Whitten, 1968), whereas, among certain groups of mammals, such as mice, the odor of a strange male can act to terminate the pregnancy of the female (Bronson et al. 1969). Because these secretions are sexual and can induce ovulation, and serve to attract potential impregnator, they are also perceived as most pleasing, during ovulation, by the human male. Indeed, males rate the odor of vaginal secretions as most pleasant when they are taken from an ovulating female (Doty, 1985).
In addition, both males and females, are effected by pheromones and the olfactory secretions of their children and their mates (Davis & Porter, 2014; Doty, 1985; Kaitz et al., 1987; Porter et al., 1986). Like insects and other animals, scent promotes individual and gender recognition, can determine caste assignment and social and economic status, as well as kinship, group, and maternal identity. For example, a breast-fed human infant is able to distinguish by smell, the breast of his mother vs that of another lactating female (Davis & Porter, 2014; Doty, 1985). Conversely, even with as little as 10 minutes of exposure to their newborn, mothers are able to identify the smell of their baby vs that of another infant (Kaitz et al., 1987). In fact, even fathers, as well as aunts and grandmothers are able to identify, by smell, a garment worn by genetically related vs unrelated infants including garments worn by the biological mother (Porter et al., 1986).
Hence, even among humans, olfaction exerts a profound effect on emotion and can signal not only sexual status, but personal identity and associated aspects of memory. These are all functions mediated by the limbic system, which in turn evolved in response to olfactory input from the olfactory bulbs and vomeronasal organ. Presumably it is because the human limbic system has become functionally organized to process these signals that it has disposed of the vomeronasal organ which has become vestigial. Thus, in humans, and as is possibly the case with adult monkeys, and apes, having been organized by the vomeronasal organ, the limbic system, in conjuction with the main olfactory system appears to continue to engage pheromonal analysis. In fact, just as the vomeronasal organ responds to phermones, so to does the olfactory bulb even in creatures that possess a vomeronasal organ (Dorries, et al., 1996).
Moreover, the limbic system (Bakker et al., 1996) including the orbital frontal lobe (Munari & Bancaud, 1992; Zatarre et al., 1992) has not only become organized to respond to olfactory/pheromonal molecules, but has become adapted to utilizing similar molecules as neurotransmitters, and has become organized so as to promote the secretion of a variety of pheromones which serve different signalling functions, such as sexual availability (Carde & Minks, 1996).
Being an evolutionary derivative of the olfactory system (as will be detailed below), and the continued dependence of the limbic system on olfactory input, also explains why monkeys, apes and humans are profoundly effected by odors, and why they may become depressed, and lose their appetite and sex drive if deprived of their sense of smell. Indeed, the major role of the human limbic system in olfaction and associated affective-memory functions, also explains why profound memory loss, such as the case with Alzheimer's disease, is also associated with loss of the sense of smell and the inability to recognize friends or family.
THE OLFACTORY LIMBIC FOREBRAIN
There is no consensus as to the evolutionary origins of the forebrain. However, it is believed by many that the forebrain is in large part an olfactory derivative and/or evolved in response to the increasing importance of olfactory input (Gloor, 1997; Herrick, 1925; Nieuwenhuys & Meek, 2015ab). Thus, when animals began to dwell upon the surface of the odorous perfumed earth and olfactory cues assumed increased importance, the forebrain not only began to expand but was dominated by the olfactory system (Haberly 2015; Smeet, 2015).
It is evident, at least from an examination of ancient endocasts, that cartilaginous and armored fish, who swam the seas over 300 million years ago possessed a forebrain which appears to be of at least equal size to the diencephalon and brainstem (Smeets, 2015). However, when the olfatory bulb and tract are included, it is apparent that almost half the brain is dominated by the forebrain (see Figure ). Similarly, although other ancient species, such as jawless fish, possessed a rather small forebrain, if the olfactory system is included, the forebrain becomes the dominant brain structure--at least in gross size. The forebrain of these and other ancient creatures is not only an extension of the olfactory system but is dominated by olfactory input.
Cartilaginous "fish" such as sharks, are considered a "living fossil" and first began to swim the seas 450 million years--just 50 million years after the close of the great Cambrain Explosion of Life. Dissection of the shark brain reveals a large forebrain and large olfactory bulbs (see Figure ). The majority of the shark forebrain consists of a dorsal and ventral amygdala-striatum (also referred to as the striatoamygdaloid gray) as well as a medial-posterior hippocampal-striatum (also referred to as the medial pallium). Thus the amygdala and striatum are enmeshed forming a dorsal lateral/ventral lobe, and the medial posterior striatum-hippocampus are ensmeshed forming a medial core, as is also evident in cyclostromes, fish, and (to a much lesser degree) in tailed amphibians (urodela). The dorsal/ventral amygdala/striatum and the medial/posterior hippocampus/striatum form a composite allocortical structure, with the former dominated by the olfactory system and the latter dominated by the visual system (Haberly, 2015; Smeets, 2015; Ulinski, 2015).
As animals emerged from the sea and began to live on dry land, the amygdala and striatum were pushed apart. With the evolution of amphibians (the tailless anura) the amygdala and the striatum (and much later the hippocampus and striatum) became separate structures (Herrick, 1925; Nieuwenhuys & Meek, 2015ab; Stephan & Andy, 1977; Ulinksi, 2015). In part, this separation was induced due to the increased motor demands of living on dry land, such that the motor aspects of the striatum began to increasingly differentiate and to evolve in response to and in order to meet these new motoric needs. The dorsal aspect became a rudimentary dorsal striatum, and the ventral aspect became the ventral (limbic) striatum, whereas what would become the hippocampus remained dorsal-medially located and part of the medial striatum (Gloor, 1997); i.e. the light and visually sensitive medial pallium.
Living on dry land placed new demands on the visual, auditory, and olfactory system. Hence, the amygdala, hippocampus, and the olfactory system in particular began to differentiate and evolve in response to this exceedingly complex olfactory, visual, and auditory environment.
Specifically, it appears (as based on comparative neuroanatomy) that the ventral aspect of the amygdala-striatum began to separate forming a distinct amygdala segment which remained ventrally located, and which extended along the ventricular floor, merging with the preoptic area as is the case in anurans (tailless amphibians). In mammals and humans, this ventral segment has twisted and rotated and has been pushed anteriorally, becoming the medial amygdala. Yet another segment initially remained somewhat dorsally situated (though still connected with the medial amygdala), becoming over the course of evolution (and as it rotated, shifted position and was pushed ventrally and anteriorally) the lateral amygdala. With the evolution of amphibians and reptiles, much of the amygdala had been displaced and was now ventrally located, whereas the hippocampus remained medially-dorsally situated (Gloor, 1997; Stephan & Andy, 1977; Ulinski, 2015).
One of the most important factors in the evolution of the amygdala, hippocampus, and the limbic system, was the evolution and development of a second olfactory system as represented by the vomeronasal organ which is organized for analyzing pheromones and water soluble chemical proteins such as those related to sexual status. Whereas the olfactory bulbs had projected predominantly to the amygdala-striatum, the vomeronasal organ transmitted directly to the now completely distinct amygdala via the accessory olfactory bulb and accessory olfactory tract, as well as to the hypothalamus and the hippocampal-striatum.
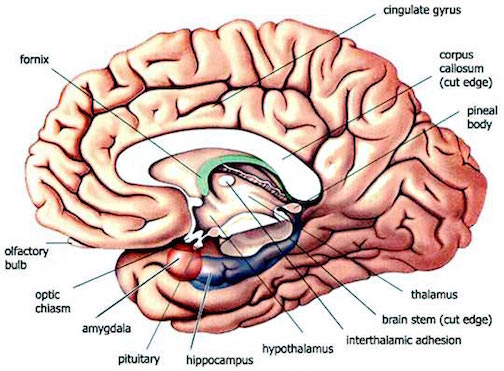
As the amygdala became reorganized to process this secondary olfactory input, the main olfactory system also increased in size and increased its projections to the amygdala, and the hippocampus and hypothalamus (Stephan & Andy, 1977). Hence, all three limbic structures were directly influenced by olfactory/pheromonal cues, the hypothalamus responding emotionally, sexually, or with hunger, and the hippocampus comparing this data to previous impressions and related visual input, and then storing this information in memory. As the hippocampus was also receiving visual input, it began to expand, becoming increasingly allocortical, forming (along with the amygdala) the piriform lobe.
As the amygdala became dominated by both olfactory systems and was now responding not only to olfactory signals, but pheromonal signals as transmitted by the vomeronasal organ (e.g., Bakker et al., 1996; Dudley et al., 1992), it grew in size and began to differentiate even further, forming, in conjunction with the hippocampus, the three layered allocortical piriform lobe (Haberly, 2015; Ulinksi, 2015). Moreover, like the hippocampus the amygdala began to increasingly received visual as well as auditory input. Hence, the piriform lobe (which contains the amygdala and hippocampus), although olfactory-dominated, also began to increasingly receive auditory and visual input, which induced additional expansion and differentiation, thus promoting the evolution of the four to five layered mesocortex (Gloor, 1997).
To recapitulate, this great expansion and reorganization of the amygdala/hippocampal-striatum was initiated with the evolution of the first amphibians. The first amphibians probably evolved from a variety of precursor forms, including the freshwater dwelling rhipidistians (a branch of the crossopterygians). This was 350 to 400 million years ago, during the Devonian (Caroll, 1988). The amphibian brain shares basic similarities with the brain of the fish. However, as they became adapted to living on land, the dorsal amygdala-striatum began to expand in response to increased olfactory, visual and auditory input, and in consequence the amygdala began to split off (Stephan & Andy, 1977)--a consequence also of penetrating fiber pathways directed to it and the striatum by the visual and auditory systems and the enlarged olfactory system.
Living in a perfumed world of smell, the olfactory cortex began to expand its projections to and representation within the forebrain. As the environment acts on gene selection, this world of smell also acted on gene selection, thus giving rise to an accessory olfactory lobe, and the vomeronasal organ which were specialized for analyzing these additional and more varied and complex olfactory sensations, including those chemical messengers referred to as pheromones.
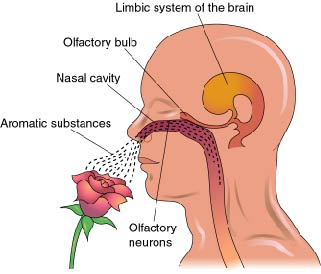
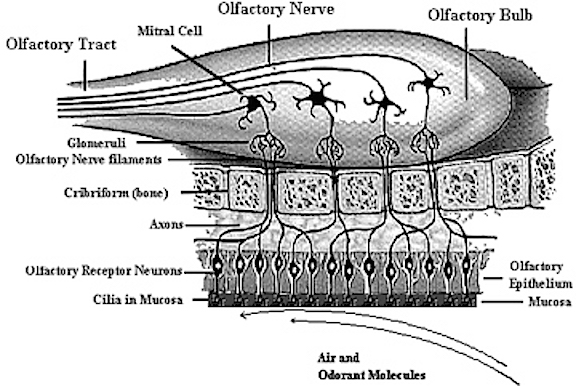
Fish, sharks, and other non-mammalian denizens of the sea, do not possess a vomeronasal organ (Smeets, 2015). Rather, these creatures have only two chemosensory systems, taste and olfaction which they employ to detect motivationally significant stimuli such as the presence of food, a mating partner, or a predator. Thus, the evolution of the vomeronasal organ was a completely novel development requiring major alterations in the structure and organization of the forebrain.
As the olfactory system more than doubled in size and importance, it also increased its privileged access to the telencephalon and further acted to push apart the amygdala and striatum, thus creating a completely separate amygdala (Stephan & Andy, 1977). Through this reorganized and more emotionally-sensitive amygdala, and via the nascent vomeronasal organ and enlarged olfactory system, amphibians were provided the ability to analyze distant as well as old and recent olfactory and pheromonal cues that could not be detected by main olfactory system.
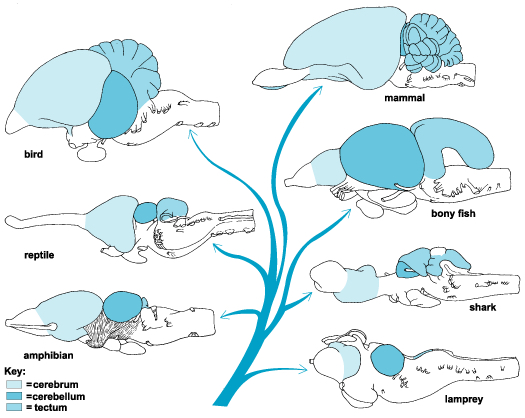
For example, via the detection of pheromones the organism could determine the intent and social-emotional status of conspecies who may have passed by hours or even days before, as well as signal and leave chemical messages conveying it's own intent, motivation, social position, and/or sexual availability (Ackerman 2015; Blum 1985; Mayer & Mankin 2015; Michael & Keverne, 1974; Tamaki 2015; Wilson, 1980). Moreover, not just food and potential mates, but status, aggressive vs submissive intent, sickness and health, and individual identity could be easily ascertained as well as signaled via the secretion and perception of pheromonal signals, including those that could instruct in a step-wise fashion, complex behaviors including those pertaining to sex; e.g. approach, mount, insert penis, thrust, and thrust again.
In fact, just as these pheomonal secretions can trigger reflexive sexual behavior in most species, direct electrical activation of these limbic structures (e.g. the amygdala, hypothalamus) can produce involuntary sexual responses including ovulation, uterine contractions, penil erection, thrusting, ejaculation and orgasm (Backman & Rossel, 2014; Currier et al., 1971; Freemon & Nevis,1969; Warneke, 2016; Remillard et al., 2003; Shealy & Peel, 1957).
With the evolution of reptiles the vomeronasal organ had also become well differentiated. The amygdala also became organized so as to process and analyze external olfactory and pheromonal stimuli and to determine its possible social, emotional, motivational, and sexual significance--a capacity that quickly came to include the ability to process visual and auditory stimuli in a likewise fashion (Gloor, 1997). That is, capacities that evolved in response to olfactory input could now be used for analyzing visual and auditory input. Moreover, the amygdala and the hippocampus began to store this information in memory, so as to make comparisons with previous impressions, and could relay these impressions to each other and to other forebrain structures for additional analysis.
As noted, mesocortex also began to evolve, forming the four then five layers which began to coat the outer skin of the hippocampus. In consequence, olfactory and many other forms of input became redirected to the hippocampal mesocortical shroud which could process this information before transmitting it to the hippocampus--a shroud which eventually began the 7 to 8 layered entorhinal cortex (e.g. Braak & Braak, 2012; Ramon y Cajal, 1902/1955; Rose, 1926). . Through the interactions of the entorhinal cortex and hippocampus, and in response to visual and olfactory input, the hippocampus gained the ability to integrate, learn and remember these attributes including their location in visual-olfactory space. The hippocampus became increasingly able to employ olfactory and visual cues in order to form a visual-olfactory map of the environment.
The amygdala also began integrating and storing these cues in memory. Moreover, it was able to act on these signals through connections still maintained with the striatum. Hence, just as the vomeronasal organ and olfactory system are involved in mediating the classic four "fs" feeding, fighting, fleeing, and sexual activity, as well as promoting social behavior; i.e. aggregating (Halpern, 1987) the amygdala became similarly organized and also became increasingly involved in regulating reproductive activities and in controlling the affective aspects of motor behavior via the striatum.
THE EVOLUTION OF THE CIRCUITRY OF THE OLFACTORY LIMBIC SYSTEM
The vomeronasal organ also projects to the hypothalamus. The hypothalamus, therefore, also became increasingly responsive to pheromonal cues, especially in regard to sex, aggression, and feeding behaviors. In consequence, the connections between the hypothalamus and amygdala were also increased so that they could engage in coordinated action.
Likewise, since the visually responsive hippocampus/entorhinal cortex also received olfactory input, the connections between the amygdala and hippocampus were significantly expanded, and the hippocampus and hypothalamus also strengthened interconnections, thus forming the initial core of the limbic system which relied on the striatum and brainstem to act on its olfactory-triggered desires, urges and needs.
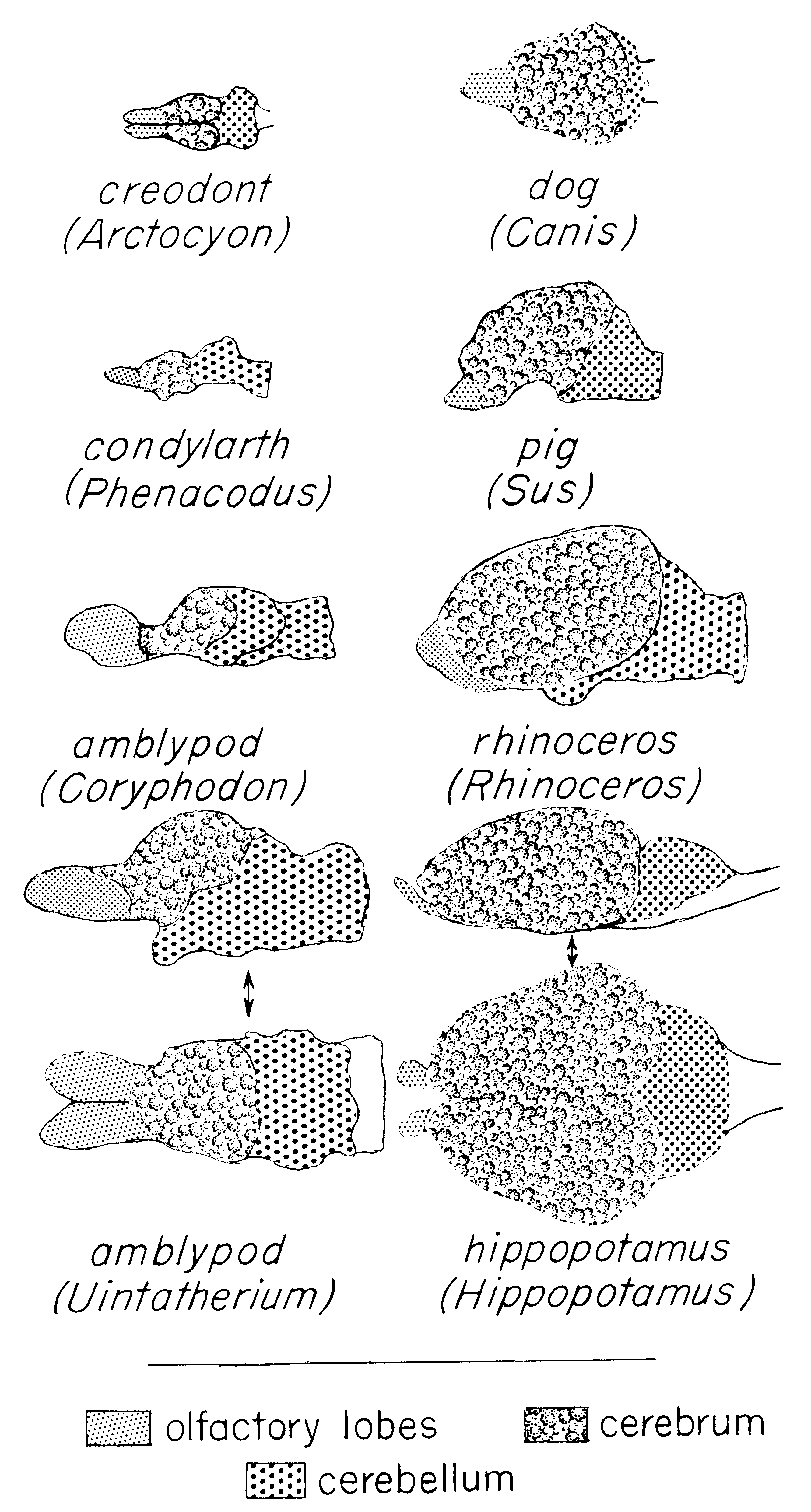
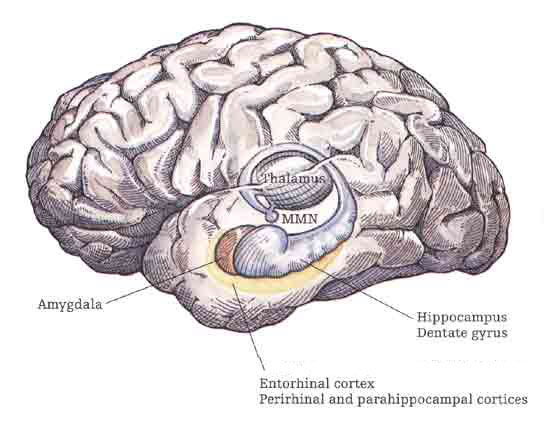
Now bound together in a common olfactory-limbic purpose, these structures could act in a coordinated fashion. The amygdala, upon detecting an emotionally significant stimulus, could act on the hippocampus, and together they could learn or generate appropriate memories. Both structures could also act on the hypothalamus so as to promote the generation of core emotional reactions including the secretion of necessary hormones and peptides. These structures could also act on the striatum so that appropriate behaviors related to feeding, fighting, fleeing, of sexual activity could take place.
The amygdala and hippocampus in particular, were also able to create an olfactory map of external reality and could not relate this to internal reality thus creating a separate internal and external reality, which in turn gave rise to personal reality, and an individual identity that was separate from the world and other creatures. Indeed, through olfactory cues, scent marking, an animal is able to advertise not only its personal, individual identity and status, but its personal possessions, such as its territory or sex partner.
THE EVOLUTION OF THE PIRIFORM LOBE, SEPTAL NUCLEI, AND CINGULATE
With the evolution of repto-mammals, over 250 million years ago, and then therapsids 200 to 150 million years ago (e.g. Probainognathus from the triassic followed by Periptychus from the Paleocene), the olfactory bulb greatly expanded as did the piriform lobe (Quiroga, 1980; Ulinksi, 2015) which came to dominate and form the largest structure (or lobe) of the forebrain. Even in primitive mammals, the piriform lobe and the other primary olfactory structures, forms the largest portion of the hemisphere including much of the lateral surface.
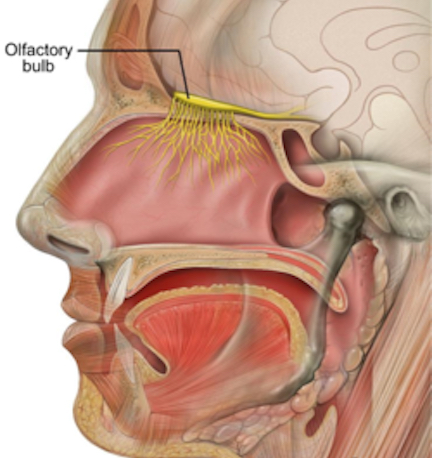
With the evolution of repto-mammals the medial portions of the brain still contained the dorsal medial hippocampus which in turn became slowly transformed so as to analyze and process the increased level of sensory input being received. Like the amygdala the hippocampus was also being pushed and stretched in different directions, the medial portions (through which interconnections with the hypothalamus were maintained) becoming tissue thin, and the ventral aspects becoming elongated. However, in this regard, the hippocampus had already begun to contribute to the evolution not only of the medial walls of the hemisphere, but to the emergence of the septum pelucidum and thus the septal nuclei which was simultaneously emerging and being yanked out of the hypothalamus by the departing hippocampus.
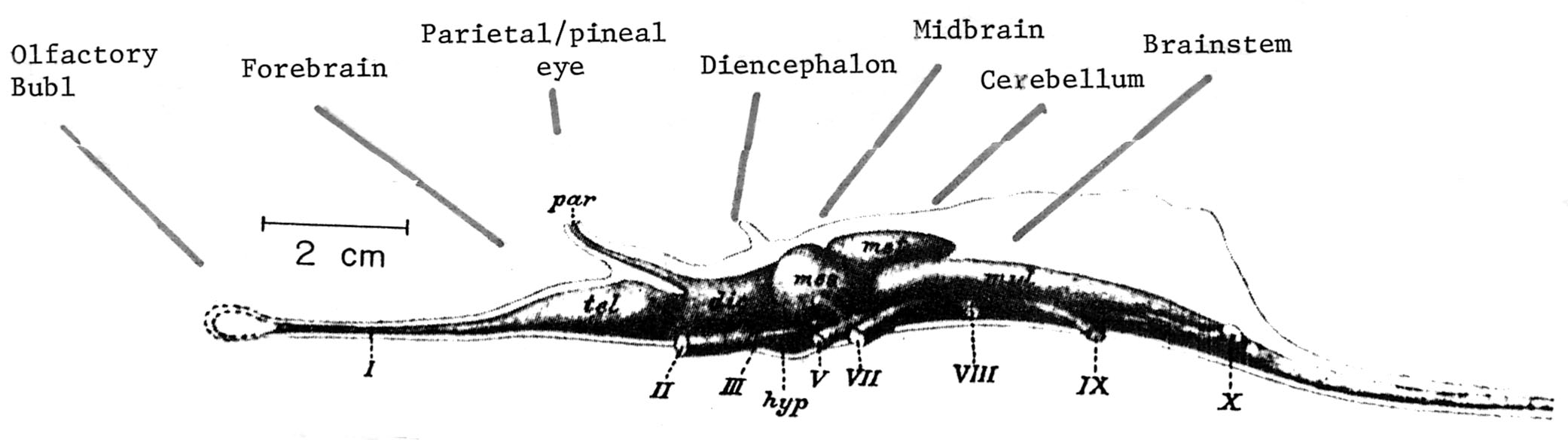
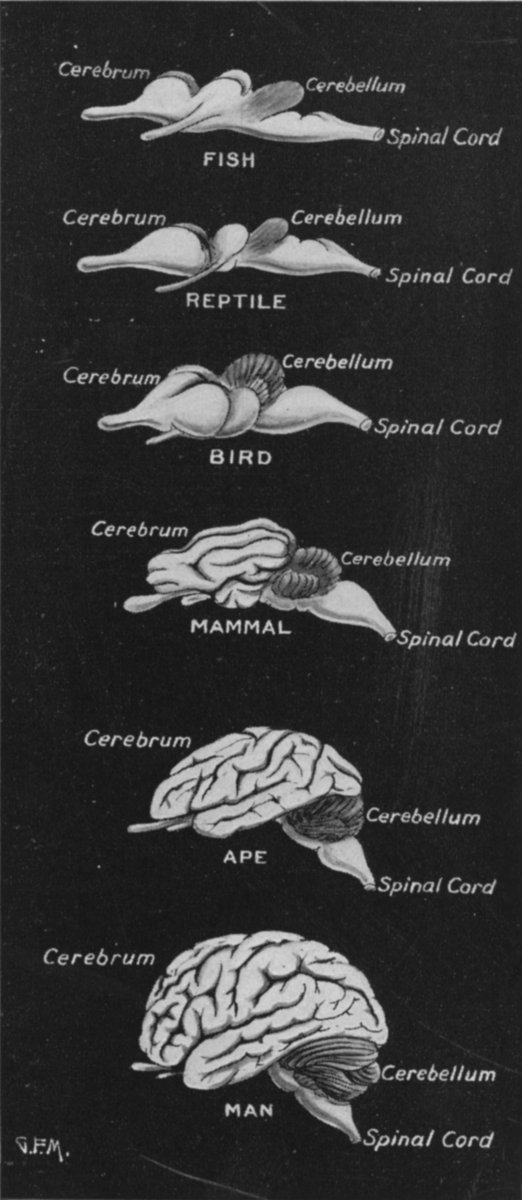
Over the ensuing 100 million years, and with the evolution of the therapsids, the dorsal-medial three layered allocortex was becoming four then five layered mesocortex, thus forming what would become the cingulate gyrus (MacLean, 2015). With the growth of cingulate cortex, the hippocampus was further dislodged and became elongated and stretched arc-wise, thereby forming dorsal-medial and ventral components. Pushing and dragging the emerging entorhinal cortex before and after it, the ventral most aspect of the hippocampus also carried with it the posterior portion of the piriform lobe which began to collide with the amygdala and the anterior portion of the piriform lobe; the collision taking place at the junction of the uncus.
As based on endocasts and comparisons to modern "living fossils" it appears that in response to increased olfactory, visual, and auditory input (around 150 million years ago) that the hippocampal half of the piriform lobe continued to sprout mesocortex which in turn would become entorhinal cortex, which it carried with it as it was pushed ventrally along with the amygdala (that is, due to continued expansion of the brain). Likewise, it appears that the amygdala began to litteraly spin out mesocortex which began to overlay portions of the allocortex. In consequence, over the ensuing 50 million years, the brain consisted not only of allocortex and mesocortex, but a combination of the two, thereby creating the six to seven layered entorhinal cortex and neocortex.
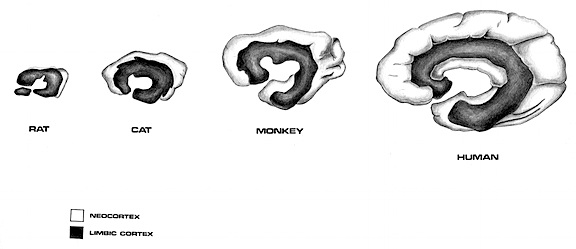
Hence, by 100,000 years ago, the piriform lobe and cingulate gyrus appear to have been capped by a small nub of neocortex (Quiroga, 1980). Over the next fifty million years this neocortical cap soon became a shroud and enveloped the hemispheres of the telencephalon and the right and left piriform lobe, thus forming a very rudimentary temporal lobe--a structure which consists of allocortex, mesocortex, entorhinal cortex, and neocortex and which contains the amygdala and hippocampus, the core components of the limbic system.
During the end of the therapsid rein, some 150-100 million years ago, and during the therapsid mammalian transition, the brain begins to resemble that of a opossum or hedghog-- as is evident based on endocasts of later appearing therapsids, e.g. Phenocodus. Thus the lissencephalic surface area of the brain consisted of allocortex, mesocortex, entorhinal cortex, and neocortex. However, in the opossum, the piriform lobe continues to make up almost two thirds of the hemisphere, and in insectivores, half of the hemisphere consists of olfactory structures. Hence, with the emergence of mammals, the cerebrum was still dominated by the olfactory system.
It is only with the demise of the dinosaurs, which enabled mammals to gain dominion over the day as well as the night, and thus with the ensuing evolution of primates, monkeys and apes in particular, that the olfactory dominance came to be challenged and supplanted by visual and auditory input. And with the evolution of monkeys and apes, the olfactory input to the amygdala was also reduced whereas visual and auditory input became dominant. Likewise, olfactory input into the hippocampus was almost completely eliminated, being directed instead to the entorhinal cortex. Hence, the hippocampus also became dominated by visual input (and less so auditory input).
SUMMARY
Hence, in summary, the great expansion of the brain was a direct consequence of the increased importance of the olfactory system, with the brain expanding to accommodate olfactory needs. Much of the forebrain, therefore, is a derivative of the olfactory system which in turn induced the differentiation and reorganization of the amygdala-striatum thus creating an amygdala and striatum and contributing to the evolution of the hippocampus.
It is noteworthy that the original unity of the amygdala-striatum and its eventual separation is also repeated over the course of embryological development--yet another of the almost innumerable examples of ontogeny replicating phylogeny. For example, around the sixth week of fetal development immature neuroblasts migrate in massive numbers from the ventricular lining, and congregate in the more caudal portion of the emerging forebrain, thus forming an arc shaped "striatal ridge" from which the primordial amygdala will emerge (Gilles et al., 2003; Humphrey, 1968). However, approximately one week after the formation of the amygdala, this primordial amygdala-striatum begins to differentiate and balloon outward to create the striatum. That is, both the striatum and amygdala are derived from the arc shaped "striatal ridge," the caudal portion giving rise to the primordial amgydala at about the 6th week of gestation, and the basal portion later giving rise to the primordial striatum which initially overlies and is contiguous with the amygdala (Gilles et al., 2003; Humphrey, 1968). Over the ensuing weeks, these structures are pushed further apart thus again, replicating phylogeny. Moreover, just as olfactory influences are important in the evolutionary differentiation of these structures, so to are olfactory influences important in embryology and the formation and development of the forebrain (Joseph, 2008d).
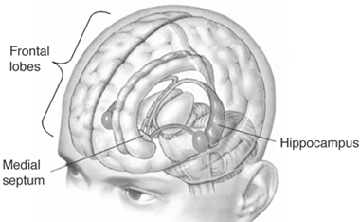
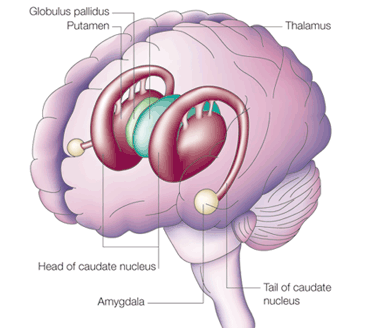
For much of neurological evolution, the olfactory-amygdala-hippocampus- hypothalamus dominated forebrain functioning--as is evident from the size of the piriform lobe. Moreover, for much of early mammalian evolution, the olfactory-forebrain continued to dominate cerebral and cognitive functioning (Gloor, 1997; Haberly 2015). According to Gloor (1997, p. 54), "the earliest development in mammalian brain evolution seems to have been a growth spurt of olfactory telencephalon. The isocortex in these forms sat like a small cap on top of a very large olfactory telencephalon."
However, over the course of later mammalian evolution and as these creatures gained complete dominion over much of the planet, the neocortex began to expand at a rapid rate (Stephan & Andy, 1977). In fact, when comparing the brains of "living fossils" such as insectivores with that of humans, it appears that the neocortex expanded by a factor of 156 (even when taking into account differences in body size), whereas allocortex and mesocortex and all associated olfactory-limbic structures developed at a much reduced rate such that the hippocampus and septum are only 4 times larger and the amygdala is 6 times larger when comparing humans to insectivores, whereas the olfactory bulb is 40 times smaller (Stephan & Andy, 1969; Stephan, 2003). Indeed, not only does the olfactory bulb shrink with the evolution of humans, but the vomeronasal system has become vestigial.
THE OLFACTORY LIMBIC SYSTEM: SUMMARY AND FUNCTIONAL OVERVIEW
Although the olfactory system in humans and hominoids is allometrically reduced in size as compared to macrosomatic animals, it remains similarly organized and continues to exert a profound influence on human behavior. Moreover, structures such as the human amygdala, hypothalamus, and hippocampus, although seemingly freed of olfactory dominance, continue to process and react to sensory-affective stimuli in a manner originally dictated and signaled by the olfactory system.
The olfactory system, for example, is capable of learning and forming memories (Brennan et al., 2015; Woo et al., 1987), and specific "positive" odors (e.g. peppermint) have been reported to improve learning and memory (Baron, 2015; Ehrlichman & Bastone 1992). These same learning-related cognitive attributes are also associated with and dominated by the amygdala and hippocampus/entorhinal cortex in interaction with the frontal lobes and dorsal medial nucleus of the thalamus (see chapters 14, 19)--forebrain structures which receive direct olfactory input and which become activated by olfactory stimuli (Bakker et al., 1996; Dudley et al., 1992; Munari & Bancaud, 1992; Zatarre et al., 1992) and which when injured result in profound memory loss (Baron et al., 2004). Moreover, many of these same forebrain-olfactory targets display genetic changes and increased protein expression in response to olfactory signals (Bakker et al., 1996; Dudley et al., 1992); activities also associated with learning and memory.
Olfactory stimuli, in humans, are capable of establishing profound memories that may be recalled a life time after they were formed. Indeed, anecdotally, in literature (Marcel Proust, Remembrance of Things Past), and in numerous studies it has been reported and demonstrated that various odors effect learning and memory and have the capability of triggering vivid recollections of some far away and past event (Herz & Cupchick, 2012; Hirsh 2013). For example, when college students were presented with various scents, they commonly reported highly personal, emotional memories, many of which were from early childhood (Herz & Cupchick, 1992).
Likewise, activation of the amygdala can provoke profound emotional memories, many of which are from childhood (Gloor, 1997; Halgren, 1992; Penfield & Perot, 196), whereas destruction of the amygdala and hippocampus (Eichenbaum, et al., 1993; Milner, 1966; Milner et al., 1968; Squire, 1992; Terzian & Ore, 1955) and the right frontal lobe (Baron et al., 2004) can abolish not only the ability to form new memories or recall those formed years before (Milner et al., 1968), but to smell and discriminate odors. For example, the famous bilateral temporal lobectomy patient, H.M., "when shown a lemon he recognized it readily by sight, but when sniffing it he remarked: Funny, it doesn't smell like a lemon" (Gloor, 1997). Likewise, the right (orbital) frontal lobe is not only a major target and dominant (over the left) for olfactory sensations (Munari & Bancaud, 1992; Zatarre et al., 2012) but becomes activated during learning (Brewer et al., 2008) and when recalling autobiographic information (Dolan et al., 1997; Frith & Dolan, 1997; Kapur et al., 2005; Tulving et al., 2004), and if injured can result in profound memory loss (Baron et al., 2004), and olfactory discrimination deficits (Zatorre & Jones-Gotman, 2014).
Likewise, individuals suffering from severe memory loss and Alzheimer disease have been repeatedly found to have abnormalities in the hippocampus, amygdala, and substantia innominata/limbic striatum (see chapter 16), and tend to suffer from severe odor detection deficits (Doty 1987, 2014). Indeed, loss of smell may be one of the earliest indicators of Alzheimer's disease onset. Moreover, just as olfactory molecules enables humans (and other animals) to determine and recognize kinship (Davis & Porter, 2014; Doty, 1985; Kaitz et al., 1987; Porter et al., 1986), patients with Alzheimer's disease commonly suffer from an inability to recognize friends and loved ones. In the advanced stages of the disease, they not only fail to recognize others, but their own personal possessions and the location of the rooms in one's house and even the house itself--all of which may be permeated with the subtle pheromonal secretions of the occupants--pheromones which can no longer be detected.
Odors and pheromones, are commonly employed to signify ownership and one's territory and to determine and localize objects and individuals in space through the creation of an olfactory map and by the detection of associated odors. Although the hippocampus no longer receives direct olfactory input (which is relayed via the amygdala and entorhinal cortex), the hippocampus has retained the ability to compute and localize the creature's position as well as that of other creatures and objects in space (Nadel, 2014; O'Keefe, 1976; O'Keefe & Nadel 1974; Wilson & McNaughton, 1993), and if injured, the ability to remember and discriminate olfactory stimuli is impaired (Zatorre & Jones-Gottman, 2014), as is learning and memory. Learning, memory and cognitive-spatial positional analysis are attributes characteristic of the modern amygdala and hippocampus (Enbert & Bonhoeffer, 1999; Gloor, 1997; Halgren, 1992; LeDoux, 1996; Nunn et al., 1999; Xu et al., 2008), including (especially in the case of the amygdala) the ability to recognize friend's, loved one's and to feel love and affection toward them (Lilly et al., 2003; Marlowe et al., 1975; Terzian & Ore, 1955) --an olfactory derived and supported attribute.
It is rather obvious that olfaction continues to exert a profound influence over human behaviors, and maintains a leading role in the signaling of significant information. One need only suffer a severe cold in order to appreciate the dominant function of smell in the ability to fully detect and appreciate the flavor of food and thus experience pleasure in eating; and they need only be approached by the long unwashed in search of spare change, to experience the repellent power of odor. Humans are in fact capable of detecting and remembering as many as 10,000 odors. About 1% of the human genetic blue print remains devoted to olfactory functioning and there are almost 1,000 different genes which are responsible for the creation of odor detecting nerve cells.

Moreover, humans obviously secrete odors and these secretions signify personal possessions and individual identity, sickness and health, and sexual availability. The limbic system is sexually differentiated (Allen et al. 2015; Bleier et al. 2012; Dorner, 1976; Gorski et al. 1978; Nishizuka & Arai, 2011; Rainbow et al. 1982), as is the olfactory system (Segovia & Guillamon, 1993) and the olfactory limbic system is especially sensitive to pheromones communicating sexual status (Winans et al., 1982) as is the human male and female (Doty, 1985; McClintock, 1971; Veith et al., 2003)--which is also why humans retain the capacity to secrete (as well as perceive) these substances.
Humans manufacture and secrete these chemical messengers in their saliva, in their sweat, through vaginal and anal secretions, through their hands and feet (thus marking what they touch and where they walk), and via scent producing glands referred to as anxillarly organs--all of which exert profound effects on the behavior of others. Hence, the abundance of artificial chemicals designed to eliminate various body fragrances, or conversely, to smell "sexy."
Anxillarly organs are located under the arms and are maximally developed among females who presumably secrete these substances to socially communicate, and to attract and entice sexual admirers--the human female is like a walking flower, enticing with her sexual perfume, potential pollinators who arrive drunk with desire. As noted, men find the vaginal smells of women most pleasant when they are at that point of their cycle when they are most likely to conceive, and like innumerable other animals, the human male may even drink and eat up these secretions through cunnilingus.
In most species the effects of many pheromones on the limbic system and sexual behavior are almost reflexive if not overpowering. In response to a sex pheromone being actively secreted by a nearby receptive female, the vast majority of male insects, cats, dogs, etc., are compelled to respond and to travel long distances in order to mate, and they will persist in their attempts even when actively rebuffed or there is a threat of being killed or eaten. In this regard it is noteworthy that although the (Western) human female may labor to scrub away her own natural sexual perfumes, she nevertheless continues to serve her olfactory limbic system, and its own peculiar motivating desires, by inundating herself with a variety of perfumes--many of which are extracted from the sex glands of other animals, or flowering plants-- the purpose of which is not merely to smell pleasant, but to indicate sexual availability and to arouse the members of the opposite sex. Again, this is also why some of the most popular and expensive perfumes have such obvious names: "My Sin," "Tabu," "Decadence," "Opium," "Indiscretion."
In general, male humans are able to resist reflexively reacting to these obvious olfactory sexual signals (even if exuded by a high heeled, perfumed, mini-skirted beauty dressed in "f---- me" attire), and this is accomplished not only by the threat of social and legal sanctions, but via frontal lobe inhibition (which receives direct and indirect olfactory projections). Indeed, the frontal lobes--the senior executive of the brain and personality--functions in part by inhibiting the limbic system (see chapter 13), and in fact evolved and increased in size in parallel with the temporal lobe (within which are located the core structures of the limbic system). However, because the human male may still feel impelled by his limbic system to respond, but due to his inhibiting frontal lobe and thanks to six to seven layered neocortex, he may instead rely on socially acceptable strategies to satisfy these limbic lusts. He ask her for a date, buys her sweet smelling candy or chocolate etc., so that what is desired might be acquired.
Nevertheless, among humans, despite the tremendous expansion in neocortex, particularly within the frontal lobe, emotional upheavals frequently occur, often with murderous or sexually inappropriate consequences. Among humans, these old limbic and rhinencephalic influences remain quite powerful and exert almost continual streams of influence which profoundly affect different aspects of our lives including not just sex and the consumption of food, but the manner in which we interact with strangers and loved one's alike. Although largely unconscious, we still react to, employ and rely upon olfactory and pheromonal molecules to communicate, the essence of which tells us about the world, and through which the world is told about us.
REFERENCES