Rhawn Joseph, Ph.D.
BrainMind.com
The Occipital lobes are association with simple and complex visual information processing which involves other cortical and subcortical regions of the brain. Visual functioning is not restricted to the occipital lobes for over half the primate neocortex is also concerned with visual functions (Reid, 1999; Zeki, 1997). In fact, although much of thalamic-visual- retinal input is directed to the primary visual receptive areas in the occipital lobe (Broadmann's area 17), visual input is also transmitted directly to other visual areas such as the temporal and parietal lobes, including signals related to rapid motion and object recognition (Haxby et al., 1991; Sereno et al. 2002; Zeki, 1997). For example, the superior parietal lobe also contains visual cortex and receives peripheral and lower visual input via the optic radiations, whereas the middle and inferior temporal lobes receive foveal and upper visual field input via these fibers (Doty, 1983; Kaas & Krubitzer 1991; Lin et al. 1982; Sereno et al. 2002).
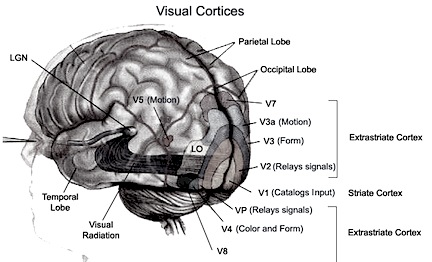
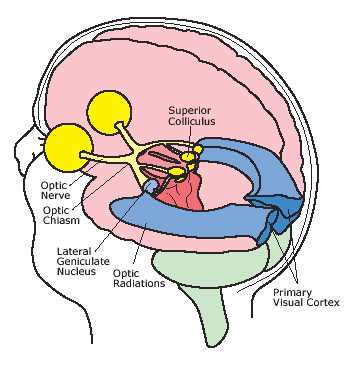
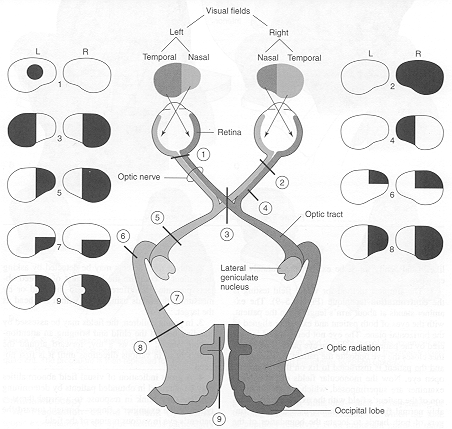
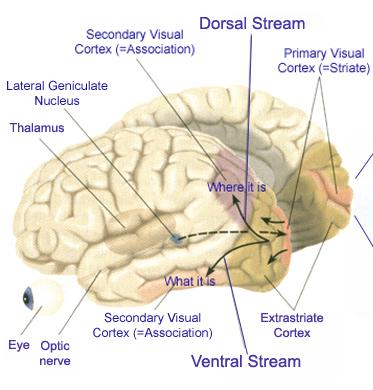
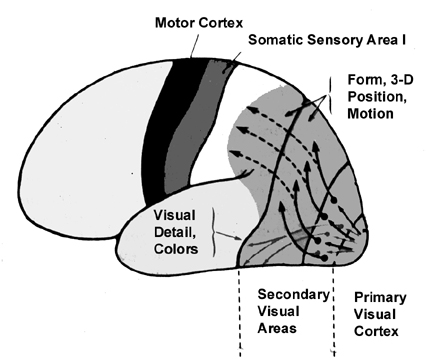
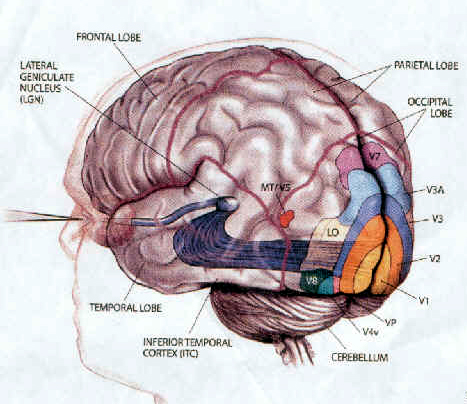
THE 'VISUAL" MIDDLE INFERIOR TEMPORAL LOBE
The middle temporal lobe, (MTL) like other structures throughout the brain, appears to be functionaly lateralized such that the right temporal region is more involved in visual and non-verbal auditory functioning, whereas the left MTL is more concerned with auditory-linguistic capabilities. Hence, whereas the auditory-linguistic zones appear to be more extensive within the left temporal lobe (and include portions of the posterior temporal region), the right MTL seems to be more associated with visual responsiveness. In general, however, the middle temporal gyrus of both hemispheres appear to contain visually responsive neurons (Beckers & Zeki 2002; Felleman & Kaas, 1974; Heit et al. 1990; Maunsell & Van Essen 1984; Selemon et al. 2014). Nevertheless, since there have been relatively few published studies of the effects of lesions or the functional capabilities of the middle temporal lobes in humans, notions regarding the extensiveness of auditory vs. visual lateralized representation are admittedly speculative.
MIDDLE AND INFERIOR TEMPORAL LOBE VISUAL FUNCTIONING
Visual cells in the MTL receive direct projections from the striate cortex, area 17 and the association area 18 (Wall et al. 1982). This area in turns projects to the MTL of the opposite hemisphere via the corpus callosum, ipsilaterally to areas 17, 18, 19, the inferior temporal and the parietal-occipital cortex (Tigges et al., 1981; Wall et al., 1982), and maintains interconnections with the pulvinar, superior colliculus and pontine nuclei of the brainstem (Walls et al., 1982). Hence, this regions has connections with areas concerned with visual and visual attentional capabilities.
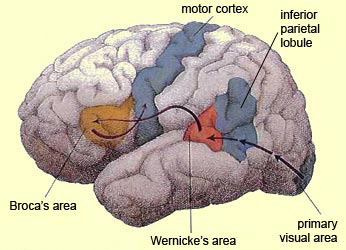
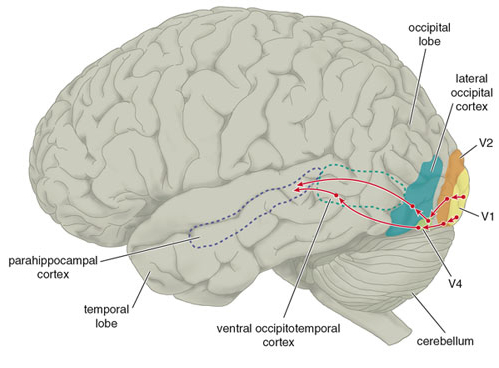
MTL neurons are sensitive to speed and direction of stimulus movement, motion, orientation, width, and disparity (Felleman & Kaas, 1984; Maunsell & Van Essen, 1983). However, most MTG neurons are not particular sensitive to the form of a stimulus--most preferring narrow stimuli (Albright et al. 1984; Maunsell & Van Essen, 1983). A large number of cells have binocular capabilities and respond to stimuli from either or both eyes. Hence, these cells are significantly involved in stereoscopic vision as well as determining distance.
Although not specialized for perception of form per se, via the combined analysis of width, orientation, speed and direction of movement these cells can process visual stimuli in a three-dimensional fashion, particularly in regard to trajectory, depth and position, i.e. if an object is near or far, approaching or withdrawing (Maunsell & Van essen, 1983).
Via its interconnections with the parietal-occipital lobe (an area involved in depth perception, visual fixation, and the visual-somesthetic guidance of movement) the MTL appears to provide visual guidance in regard to interactional object and body movement. That is, via the analysis of the flow of movement, local direction and speed, as well as the relative distances of objects, these neurons (in conjunction with area 7 cells) are important in guiding the movements of the body as well as the individuals limbs toward the object (Manunsell & Van Essen,1983).
The MTL maintains extensive interconnections with the inferior region and thus with limbic nuclei. It is probably via these connections that objects or conspecies of motivational significance can be discerned and attended to. That is, not only is the speed, movement, distance, etc. of a stimulus determined, but its emotional attributes (e.g. should it be feared, chased, eaten). Moreover, not just written words, but object recognition occurs in this area (in conjunction with the inferior temporal lobe), such that if injured, patients may suffer from agnosia (Giannokapoulos et al., 1999).
HALLUCINATIONS Complex visual hallucinations are associated with right occipital injuries, tumors, seizures, and other abnormalities. Electrical stimulation of or lesions involving areas 18 and 19 can produce complex visual hallucinations (Foerster, 1929, cited by Brodal, 1981 & Hecaen & Albert, 1978; Tarachow, 1941), such as images of men, animals, various objects and geometric figures, liliputian-type individuals, including micropsias and macropsias (see Luria, 1980; & Hecaen & Albert, 1978, for review). Sometimes objects may seem to become telescoped and far away, wheras in other situations, when approached, objects may seem to loom and become exceedingly large. Complex hallucinations are usually quite vivid and fully formed and the patient may think what he sees is a real (Hecaen & Albert, 1978). Foerester (1928, cited by Heacen & Albert, 1978) reported a ptient who hallucinated a butterfly then attempted to catch it when area 19 was elecrically stimulated. Another hallucinated a dog and then called to it, denying the possibility that it was not real. Complex hallucinations, although usually associated with tumors or abnormal activation of the visual association area, have also been reported with parietal-occipital involvement (Russell & Whitty, 1955), occipital-temporal, or inferior-temporal damage (Mullan & Penfield, 1959; Tarachow, 1941; Teuber et al., 1960), or with lesions of the occipital pole and convexity (Hecaen & Albert, 1978) Laterality. According to Hecaen and Albert (1978) based on their review of the international literature, although simple hallucinations are likely following damage to either hemisphere, complex hallucinations are usually associated with right rather than left cerebral lesions (Teuber et al., 1960; Mullan & Penfield, 1959; Hecaen & Albert, 1978).
Tumors involving the middle temporal lobe have been associated with the development of auditory and visual hallucinations, dreamy states, and alterations in emotional functioning--particularly as the lesion encroaches on the inferior regions (Luria, 1980). Electrical stimulation also initiates the development of complex hallucinations and alterations in consciousness (Penfield & Roberts, 1959).
INFERIOR TEMPORAL LOBE
As noted, it is possible to very loosely define the anterior-inferior temporal lobes as auditory cortex, although neurons in this vicinity receive visual as well as somesthetic input.. Hence, the inferior temporal lobes can respond to auditory as well as emotionally and visually significant stimuli (Gloor, 1997; Nakamura et al., 2014; Tovee et al., 2014).
That the inferior temporal lobe (ITL) processes complex auditory, visual and emotional stimuli is probably a function of it being a derivative of the amygdala (see chapter 14) as well as visual cortex (Diamond 1973; Gloor, 1997). Visual and auditory functions also reflect its reception of extensive (upper visual field) projections from the optic radiations, highly processed input from the visual association areas in the occipital lobes of both cerebral hemispheres (via the corpus callosum), and fibers from the superior colliculus by way of the pulvinar of the thalamus (Chow, 1950; Kaas & Krubitzer 1991; Kuypers et al., 1965; Previc 1990; Rocha-Miranda et al. 1975).
VISUAL CAPABILITIES & FORM RECOGNITION
Indeed, the neocortex of the ITL is specialized for receiving, analyzing, discriminating, recognizing and recalling complex visual information and is involved in attention and visually guided behavior (Gross & Graziano 2002; Gross et al. 1972; Tovee et al. 2014), including the recollection and learning of visual discriminations (Gross, 1972) and memory for objects and spatial locations (Nunn et al., 1999; Ploner et al., 2009). If the temporal lobe is injured, these functions become compromised.
For example, Kimura (1963) found that patients with right vs left temporal lobe injury were impaired when presented with overlapping nonsense shapes and then immediately tested for recognition. Likewise, Meier and French (1965), found that those with right vs left temporal lobe injuries were impaired when asked to make visual discriminations when presented with fragmented concentric circle patterns--skills which are also related to visual closure and gestalt formation. More recently, Nunn et al., (1999) found that right vs left temporal lobectomy patients were more impaired when required to remember and recognize toys and recall their location.
Cells in the ITL have very large, bilateral visual receptive fields which include the fovea, and many are sensitive to direction of stimulus movement, color, contrast, size, shape, orientation and are involved in the perception of three dimensional objects and the supramodal analysis of information already processed in the association areas (Eskander, et al. 1992; Gross & Graziano 2002; Gross, et al. 1972; Nakamura et al. 2014; Rolls, 2012; Sergent, et al.1990). Thus inferior temporal (IT) neurons appear to take part in the last stages of visual analysis for form recognition and receive terminal fibers from the primary and association visual areas (Gloor, 1997; Gross, et al. 1972; Ungerleider & Mishkin, 1982); that is, via the ventral visual stream. Indeed, a single neuron can respond to a combination of these features and many will fire selectively in response to particular shapes and faces (Eskander, et al. 1992; Gross, et al. 1972; Nakamura et al. 2014; Richmond, et al. 2013; Rolls, 1992; Sergent, et al.1990).
DENIAL OF BLINDNESS
With massive bilateral damage to the occipital lobe and the right hemisphere, patients will become "cortically blind", but due to the extensive involvement of the right half of the brain, althugh blind, they may seem initiallyindifferent regarding their condition, and report a variety of hallucinatory experiences which may be complex or elementary in form. Moreover, frequently these patients will initially deny they are blind (Redlich & Dorsey, 1945) --a condition referred to as Anton's syndrome. For example, a number of patients described by Redlich and Dorsey (1945), although bumping into furniture and unable to recognize objects held before them, invented elaborate excuses for their errors and failure to see; e.g. claiming that it is a little dark and they need their glasses, or conversely, that they see better at home. That is, these patients confabulate.
As discussed in chapter 10, these confabulatory abnormalities are sometimes due to a disconnection such that the Language Axis, failing to receive information from the visual cortex (i.e. that it cannot see), responds instead to associations from intact areas which concern "seeing". That is, the Language Axis does not know that it is blind because information concerning blindness is not being received from the proper neural channels.
It is also possible that these patients deny being blind, because subcortically they are still able to see. Hence, although at a neocortical level there is no sight, subcortically there remains an unconscious awareness of the visual world.
VISUAL AGNOSIA & ALEXIA
As based on functional imaging studies, the left medial extrastriate visual cortex becomes activated when reading or viewing words (Peterson et al., 1990), whereas the right inferior occipital lobe becomes active when looking at pictures (Price, 2007). In addition to letters, words, and pictures, complex form and object recognition is also subserved by the extra-striate visual areas in the occipital lobe, the ventral-inferior areas in particular, adjacent to the temporal lobe (Buchel et al., 1998; Haxby, et al., 2011). This has been demonstrated not only by functional imaging (Buchel et al., 1998; Price, 2007), but direct cortical recoding (Nobre et al., 2014), and electrical stimulation (Luders et al., 1986). In fact, both normal, congenitally blind, and late-blind subject display activity in this area (Buchel et al., 1998).
If injured, patients may suffer from reading and naming deficits (Rapcsak, et al., 1987); a condition referred to as phonological alexia (e.g., Miozzo & Caramazza, 2008). Moreover, patients with developmental dyslexia have been found to have abnormalities in this area (Rumsey et al., 1997). However, in addition to, or depending on the nature and exact location of the lesion, patients may suffer from agnosia.
Visual agnosia is a condition where the patient loses the capacity to visually recognize objects, although visual sensory functioning is largely normal. This condition often arises with lesions involving the inferior medial occipital lobe. In general, objects are detected but they lose the ability to evoke meaning and cannot be correctly identified or named (Critchley, 1964; Davidoff & De Bleser 2014; Shelton et al. 2014; Teuber, 1968). The percept becomes stripped of its meaning. For example, if shown a comb, the patient might have no idea as to what it is or what it might be used for. If asked to guess they may call it a harmonica or a tiny box. If shown a pair of spectacles, they may call it a "bicycle", or "two spoons". A picture of a dial telephone may be described a a "Clock", etc. (Luria, 1980)
Many patients are unable to sort pictures or objects into categories or match pictures with the actual object such that there appears to be a deficit in the ability to not only recognize but to classify visual percepts (Hecaen, et al. 1974). Moreover, in severe cases they are unable to point to objects that are named.
Nevertheless, this is not a naming disorder (e.g. Davidoff & De Bleser 2014), because regardless of modality, anomics continue to have word finding and naming difficulties. In contrast, those with agnosia show enhanced recognition if an object is presented via a second intact modality (e.g. if they palpate it by hand). Thus agnosia can often be limited to a single input channel, i.e. visual vs. tactual. Moreover, If an object is used in context, recognition can be greatly enhanced (Rubens, 2013).
Some patients may complain that objects change while they are looking at them, and /or that they disapear; a condition which suggests optic ataxia. Usually, however, the deficit is conceptual rather than perceptual.
There are however, to major forms of agnosia, and different subtypes as well, which can be produced by damage to tissues mediating complex visual perception or lesions disconnecting the IPL, for example, from visual, somesthetic, or auditory input.
For example, one type of agnosia, "apperceptive visual agnosia" refers to a disturbance in perceptual and visual-motor integration, such that patients have difficulty copying or matching various objects. This latter form of agnosia has been associated with lesions to the parietal occipital cortex (Mizuno, et al., 1996), as well as to bilateral damage to the inferior-occipital cortex (Shelton, et al., 2014). For example, although they may be able to accurately describe, draw or copy various aspects of the object they are shown (Albert, et al,. 1975; Mack & Boller, 2007; Rubens & benson, 1971) they fail to correctly draw the entire object. Moreover, if asked to trace rather than copy, these patients may trace over and over the outlines of objects or drawing but cannot recognize where they started.Thus they seem unable to synthesize visual details into an integral whole (Luria, 1980). That is, patients may recognize an isolated detail (e.g. the dial of a phone) but are unable to relate it to the headset, etc. (see Shelton et al. 2014) Hence, if asked what they have been shown, they may erroneously extrapolate from the detail perceived and thus confabulate a concept. With increasing object complexity, or if surrounded by other objects, or if the object is presented in pictorial (vs. actual) form, or if unnecessary lines are drawn across the picture, the ability to recognize the object deteriorates even further (Luria, 1980; Rubens & Benson, 1971).
By contrast, associative visual agnosia, which is a deficit in naming, such that auditory equivalents cannot be matched to a visual perception, is associated with left inferior and middle temporal (area 37) occipital abnormalities (Giannokapoulos et al., 1999), which may be accompanied with alexia (Feinberg et al., 2014), as well as to lesions to and atrophy of the parietal occipital cortex (Mizuno, et al., 1996).
Agnosic individuals also often (but not always, Davidoff & De Bleser 2014) have difficulty with reading (Albert, Reches & Silverberg, 1975; Mack & Boller, 2007; Rubens & Benson, 1971) and may suffer from prosopagnosia and/or impaired color naming (Mack & Boller, 197; Shelton et al. 2014). Interestingly, in some cases visual memory be intact (Rubens, 1979).
Like alexia, agnosia can occur following lesions to the medial and deep mesial portion of the left occipial lobe (Feinberg, et al., 2014). The left inferior temporal lobe and posterior hippocampus may also be damaged in some cases (Shelton et al. 2014).
In some cases, it is likely that agnosia is due not only to tissue destruction but to tissue disconnection. That is, if the visual form recognition neurons in the temporal lobe are no longer able to receive input from the visual association areas, then this particular region becomes "cortically blind" and form recognition is prevented. However, like some other disconnection syndromes, if a different input channel is employed, i.e. if the object is verbally described or tactually explored, recognition is enhanced.
PROSOPAGNOSIA.
Prosopagnosia is a severe disturbance in the ability to recognize the faces of friends, loved ones, or pets (DeRenzi, 1986; DeRenzi, et. al., 1968; DeRenzi & Spinnler, 1966; Evans et al. 2002; Hecaen & Angelergues, 1962; Landis, et al. 1986; Levine, 1978; Whitely & Warrington, 2007l ). Some patients may in fact be unable to recognize their own face in the mirror. Nevertheless, they usually realize that a face is a face; they just don't know who the face belongs to. In this regard, prosopagnosia is not a visual agnosia as described above, where the patient cannot recognize that a chair is a chair or a clock a clock.
A number of authors have erroneously argued that prosopagnosia is due to bilateral injuries involving the inferior and medial occipital lobe visual association areas. Nevertheless, although frequently such individuals do indeed suffer from bilateral injuries, in many cases the lesions are restricted to the right hemisphere and involve the occipital and inferior temporal regions (DeRenzi, 1986; DeRenzi et. al., 1968; DeRenzi & Spinnler, 1966; Evans et al. 2002; Hecaen & Angelergues, 1962; Landis et al., 1986; Levine, 1978; Whitely & Warrington, 2007; Young et al. 2002), although frontal lesions (Braun et al. 2014), and right parietal lesions (Young et al. 1992) may also disrupt facial recognition. By contrast, prosopagnosia does not usually result from lesions restricted to the left half of the brain, whereas loss of facial recognition with frontal and parietal lesions are more likely due to visual neglect and visual scanning abnormalities.
Although prosopagnosia could be explained from a disconnection perspective, it is likely that the actual face identification neurons have been destroyed whereas neurons involved in the recognition of facial parts have been preserved.
IMPAIRED COLOR RECOGNITION
In this condition, although sometimes able to correctly name objects patients cannot correctly name, match, and identify colors or point to colors named by the examiner. No, this is not due to color blindness. Frequently individuals with color imperception also display prosopanosia (Green & Lessel, 2007; Meadows, 1974b).
DeRenzi & Spinnler (1967) found that 23& of those with right cerebral damaged and 12% of those with left sided destruction had difficulty with color matching (Lhermitte et al., 1969). On the otherhand, some investigators note that impairments of color perception are frequently secondary to bilateral inferior occiptial lobe damage (Green & Lessell, 2007; Meadows, 1974a; Shelton et al. 2014). In addition, almost 50% of those with aphasia demonstrate deficient color naming and color identification (DeRenzi & Spinnler, 1967; DeRenzi et al., 1972). However, color perception per se is largely intact among aphasic individuals.
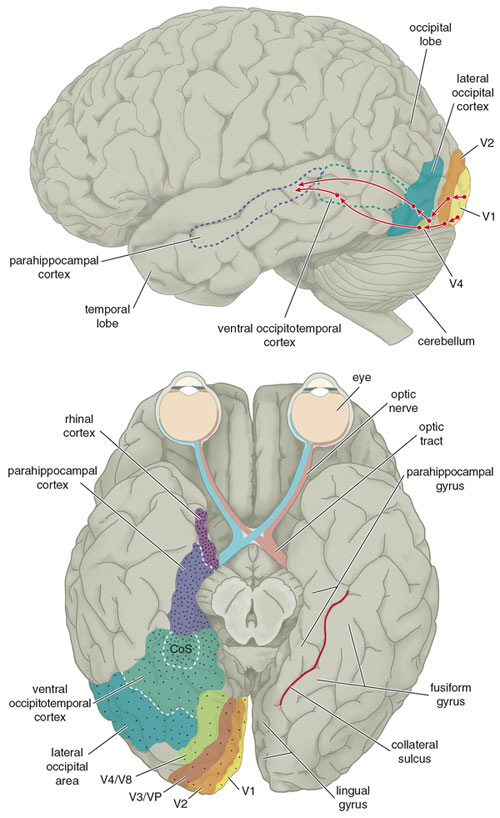
REFERENCES